النبات
مواضيع عامة في علم النبات
الجذور - السيقان - الأوراق
النباتات الوعائية واللاوعائية
البذور (مغطاة البذور - عاريات البذور)
الطحالب
النباتات الطبية
الحيوان
مواضيع عامة في علم الحيوان
علم التشريح
التنوع الإحيائي
البايلوجيا الخلوية
الأحياء المجهرية
البكتيريا
الفطريات
الطفيليات
الفايروسات
علم الأمراض
الاورام
الامراض الوراثية
الامراض المناعية
الامراض المدارية
اضطرابات الدورة الدموية
مواضيع عامة في علم الامراض
الحشرات
التقانة الإحيائية
مواضيع عامة في التقانة الإحيائية
التقنية الحيوية المكروبية
التقنية الحيوية والميكروبات
الفعاليات الحيوية
وراثة الاحياء المجهرية
تصنيف الاحياء المجهرية
الاحياء المجهرية في الطبيعة
أيض الاجهاد
التقنية الحيوية والبيئة
التقنية الحيوية والطب
التقنية الحيوية والزراعة
التقنية الحيوية والصناعة
التقنية الحيوية والطاقة
البحار والطحالب الصغيرة
عزل البروتين
هندسة الجينات
التقنية الحياتية النانوية
مفاهيم التقنية الحيوية النانوية
التراكيب النانوية والمجاهر المستخدمة في رؤيتها
تصنيع وتخليق المواد النانوية
تطبيقات التقنية النانوية والحيوية النانوية
الرقائق والمتحسسات الحيوية
المصفوفات المجهرية وحاسوب الدنا
اللقاحات
البيئة والتلوث
علم الأجنة
اعضاء التكاثر وتشكل الاعراس
الاخصاب
التشطر
العصيبة وتشكل الجسيدات
تشكل اللواحق الجنينية
تكون المعيدة وظهور الطبقات الجنينية
مقدمة لعلم الاجنة
الأحياء الجزيئي
مواضيع عامة في الاحياء الجزيئي
علم وظائف الأعضاء
الغدد
مواضيع عامة في الغدد
الغدد الصم و هرموناتها
الجسم تحت السريري
الغدة النخامية
الغدة الكظرية
الغدة التناسلية
الغدة الدرقية والجار الدرقية
الغدة البنكرياسية
الغدة الصنوبرية
مواضيع عامة في علم وظائف الاعضاء
الخلية الحيوانية
الجهاز العصبي
أعضاء الحس
الجهاز العضلي
السوائل الجسمية
الجهاز الدوري والليمف
الجهاز التنفسي
الجهاز الهضمي
الجهاز البولي
المضادات الحيوية
مواضيع عامة في المضادات الحيوية
مضادات البكتيريا
مضادات الفطريات
مضادات الطفيليات
مضادات الفايروسات
علم الخلية
الوراثة
الأحياء العامة
المناعة
التحليلات المرضية
الكيمياء الحيوية
مواضيع متنوعة أخرى
الانزيمات
Production of The Major Biofuels
المؤلف:
John M Walker and Ralph Rapley
المصدر:
Molecular Biology and Biotechnology 5th Edition
الجزء والصفحة:
23-1-2021
1988
Production of The Major Biofuels
Ethanol is currently (March 2008) being produced from over 140 commercial biorefineries throughout the USA and numerous processing plants in Brazil, yielding over 8.0 billion gallons in the USA (www.ethanolrfa. org) and nearly 4.5 billion gallons in Brazil (2007) (www.iea.org). In the USA, most of the feedstock for production of ethanol currently is corn, whereas Brazil exclusively uses sucrose from sugar cane. This latter process is the simplest as sucrose is readily metabolized by yeast to ethanol in a highly efficient mature process that provides minimal areas for improvement of the fermentation process and will not be discussed further. However, the conversion processes for corn starch-based ethanol is considerably more complex, whether it uses the simpler dry mill process or the complex corn wet milling, and both biological conversion technologies for production of ethanol from corn offer opportunities for process improvement. Due to their simplicity, dry mills comprise a majority of the corn ethanol plants.
Biodiesel use is growing rapidly in the USA using primarily soybean oil as the feedstock with additional sources coming from waste fats and oils from food processing and restaurants. Total production in the USA is only 250 million US gallons (2008), although the production plant capacity is 2.24 billion US gallons, so the production capacity greatly exceeds sales (www.biodiesel.org). In Europe, an estimated 2.3 billion US gallons of biodiesel were produced in 2007, with 84% of the biodiesel coming from canola/rapeseed sources (www.eubia.org). Over half of the production comes from Germany, with the nearest producer country, France, producing only about 30% as much biodiesel. Alteration of the crop source offers good opportunity for expansion in the USA to replace food sources such as soybean oil.
Corn Processing and Ethanol
The corn dry milling process is a simpler process than the wet mill process and has fewer unit operations. The corn is initially milled with a hammer mill or grinder to crush the corn kernels, followed by addition of water and heating of the corn slurry to liquefy the corn starch. During the heating process a thermostable a-amylase4 is added to initiate the starch hydrolysis at high temperature (90 C) and decrease microbial contaminants. This hydrolysis process generates partially hydrolyzed starch maltodextrins and little if any free glucose. Upon cooling to 50– 60 C and adjustment of the pH to about , glucoamylase is added to initiate the cleavage of single glucose units off of the starch oligomers produced by the a-amylase action. A critical process parameter is maximizing glucose generation while minimizing reversion sugars formed by reverse enzyme action as conversion approaches over 95% glucose. The corn slurry–enzyme mixture is cooled and, when the temperature reaches below 40 C, fermentation yeast, Saccharomyces cere visiae is added. This yeast is either obtained by on-site production or purchased from an inoculum producer. The fermentation continues over a few days until a majority of the starch-derived glucose is converted to ethanol, typically yielding a beer with about 9% (w/w) ethanol.
By contrast, the corn wet mill process is a true refinery process initiated by steeping the corn with water and sulfur dioxide to swell the corn and make it easily disrupted by mechanical processing. This steeping process lasts at 40–60 h and supports active lactic acid fermentation by resident Lactobacillus. Subsequently, the milling process permits the separation of the corn pericarp, corn starch, germ and soluble portion of the corn as a result of the steeping process. This steep slurry is refined by a complex series of separation processes yielding highly purified corn starch and fractions that yield corn oil after further purification, pericarp and germ residues that provide high-protein feed products. The corn steep liquor containing nearly all amino acids, minerals, other nutritional components along with typically about 50% lactic acid after extensive evaporation of the water. The highly purified corn starch is the largest product by weight and is used to produce a number of starch products such as cooking starch and derivatives destined for a variety of uses including adhesives, gums and coatings. Additionally, the starch can be further processed by a series of hydrolysis processes that include acid hydrolysis to produce a variety of specialty syrups for the baking, candy and food processing industries. Additional products employ enzymes to take advantage of their high degree of specificity that yield highly selective materials. The starch conversion process usually is initiated at high temperature (approximately 90–95 C) in a jet cooker dosed with a thermostable a-amylase, which rapidly cleaves the starch slurry to high molecular weight oligomers. Like the dry mill process, the maltodextrin syrup is then converted to over 95% glucose by the action of glucoamylase, but with much higher purity compared with the dry mill process. The glucose is purified for the incomplete hydrolysis products and is destined for various uses, including intravenous glucose, fermentation grade glucose used to produce light beer and sold to the food industry. One of the largest markets is the production of high-fructose corn syrup (HFCS), which is produced by conversion of glucose to fructose by the action of xylose/glucose isomerase, typically sold as 42% fructose, or via enrichment processes, 55% fructose HFCS. A chromatographic enrichment process is needed to attain 55% fructose due the natural enzymatic equilibrium between glucose and fructose at about 42% fructose. HFCS is sold largely as a sugar substitute, especially to the soft drinks market.
Purified glucose is highly versatile and can be used as a carbon source for a number of fermentative conversion processes. Over the last two decades, large volumes of ethanol have been produced by yeast fermentation in high purity and yield using wet mill-produced glucose. This highlights the benefit of the capital intensive wet mill process. For example, wet mill operations can produce ethanol from the glucose or divert any specified amount of the glucose stream to produce alternative higher value products. Examples of existing higher value products include antibiotics, amino acids and other food and feed additives such as vitamins. Glucose is also used to produce industrial products such as enzymes, organic acids such as citric acid and chemical intermediates such as 2,3-propanediol. This chemical intermediate is being produced by the DuPont/Tate and Lyle joint venture, located in Loudon, TN, using glucose obtained from the adjacent Tate and Lyle corn wet mill.
Biomass Conversion for Ethanol
Multifaceted bioconversion technologies are emerging for the production of ethanol from lignocellulosic biomass. A high degree of complexity is required because, unlike corn kernels, which are over 70% starch on a dry basis, biomass contains three primary structural components, 33–50% cellulose, 17–35% hemicellulose and 12–24% lignin, with the remainder being minerals, protein and other minor materials. In addition, since in Nature plants must withstand normal degradative processes as part of their survival, plant cell walls and the exterior of plant are highly resistant to enzymatic and microbial attack. As a result, over the last 30+ years a number of conversion processes have evolved that vary in complexity. The initial disruptive process needed to initiate the structural breakdown of biomass is called pretreatment and at least seven processes have been developed, ranging from highly alkaline to
acidic environments and organic solvent based. They all accomplish the partial disruption of the plant structure to allow the internal components, in particular the carbohydrates, to be exposed to enzymatic and microbial attack under controlled environments of a fermentation process. These processes vary from multi-enzyme and multi-fermentation approaches called separate hydrolysis and fermentation (SHF)to simpler simultaneous cellulose hydrolysis or saccharification and fermentation (SSF). In the SHF process, the biomass cellulose is hydrolyzed with cellulases to liberate fermentable glucose followed by a separate step for fermentation to ethanol. The SSF process combines the enzymatic hydrolysis with the fermentation, simultaneously reducing the process complexity. A natural extension is simultaneous saccharification and cofermentation (SSCF) with microorganisms that are able to covert both hexose and pentose sugars to ethanol. This process simplification culminates with the development of fermentation microorganism produces its own enzymes for cellulose hydrolysis, called consolidated bioprocessing (CBP). A fermentation technology that is the most versatile is the combination of gasification of biomass and other organic materials to a gaseous form followed by fermentation of the resulting gases to ethanol. All these conversion options have significant potential to benefit from use of advanced molecular and biotechnology analysis tools offered by systems biology tools.
1. Enzymatic Hydrolysis and Conversion
The enzymes that are typically employed in the hydrolysis of the biomass carbohydrates are cellulases including endoglucanases or β-1,4-endoglucan hydrolase (EC 3.2.1.4) and exoglucanases which are glucan 1,4-b-glucosidases (EC 3.2.1.74). An additional required enzymatic activity is 1,4-β-cellobiosidases (EC 3.2.1.91) and β-glucosidases (EC 3.2.1.21), that collectively hydrolyze cellobiose disaccharide and other oligosaccharides to glucose. These combined enzyme mixtures are fully capable of hydrolyzing both crystalline and amorphous cellulose to glucose, making it available for fermentation to ethanol.Depending on the pretreatment process used, additional enzymes may be required, such as hemicellulase enzymes such as endo-1,4-β-D-xylanase (EC 3.2.1.8) and β-xylosidase (EC 3.2.1.37), to hydrolyze hemicellulose (xylose) polymers that are poorly hydrolyzed under alkaline conditions.
Biomass sources also contain limited amounts of starch and pectin and so addition of a´ -amylase, glucoamylase and pectinase enzymes may be beneficial for selected substrates. Finally, biomass contains between 12 and 24 wt% lignin, a complex polyphenolic phenylpropanoid polymer built up from p-coumaryl, coniferyl and sinapyl alcohols, resulting in complex arrangement of p-hydroxyphenyl (H), guaiacyl (G) and syringyl (S) units.There are a number of enzymes that are known to partially degrade this complex material, including lignin peroxidase (EC 1.11.1.7), manganese peroxidase (EC 1.11.1.13) and laccase (EC 1.10.3.2), but they have not been involved in ethanol production from biomass due to cost and conversion considerations.
Enzymatic hydrolysis processes ideally produce a mixed sugar stream with predominantly glucose and xylose sugars along with a number of minor sugars such as arabinose, mannose and galactose, which collectively must be converted to ethanol by fermentation to maximize ethanol yield and specific productivity. Table .1 shows examples of specific analyses for three selected plants which shows the considerable variation
Table .1 Selected examples of detailed composition of selected biomass.
in cellulose (glucan), xylan, lignin and ash content.However, the minor sugars plus sugar acids together comprise 5–6% of the mass of biomass and should not be ignored as they contribute to ethanol production. The production of microorganisms that are able to ferment these various sugars has been the subject of active research over the last three decades and has included yeast (Saccharomyces, Pichia) and bacteria such as E coli, Zymomonas and Klebsiella.Particularly challenging has been the goal of adding the capability to ethanol-producing (ethanologen) microorganisms the ability to ferment the five carbon sugars along with glucose, since many microorganisms lack this dual fermentation capability or ferment xylose with significant diauxic lag extending the fermentation time excessively. Significant progress has been made with a number of these microorganisms and a limited number have been chosen for commercialization, although details are often scarce. In addition, through the use of genetic engineering, research efforts are under way to combine the need for hydrolytic enzymes to be synthesized by a versatile multi-sugarfermenting microorganism. Ingram’s group, using both Escherichia coli and Klebsiella, is actively pursuing the addition of cellulases to versatile ethanologens. Initial results have demonstrated the beneficial contribution of genetically adding a cellulolytic enzyme gene and capability to the genetic make-up of an ethanologen. Similar efforts are under way with yeast and the development of an improved SSF ethanologen microorganism that produces one or more required complex carbohydrase enzymes appears likely.
2 .Consolidated Bioprocessing
The possibility of the simultaneous production of hydrolytic enzymes that facilitate the degradation of complex carbohydrates to simple fermentable sugars along with fermentation to ethanol and other chemical byproducts is not new. Nature has provided anaerobic microorganisms that conduct this challenging task as a fundamental part of our ecosystem’s carbon cycle. Both mesophilic and thermophilic microorganisms are known to degrade and ferment biomass carbohydrates producing ethanol, other alcohols, organic acids and hydrogen as common byproducts of their anaerobic fermentation.
Intense research efforts have been made in a number of laboratories to harness selected individual candidate microorganisms that rapidly hydrolyze cellulose and/or hemicellulose and produce ethanol as part of their byproduct profile, through the aforementioned process of CBP.
Of particular interest have been the cellulase enzymes that facilitate the biomass cellulose breakdown. Through research over two decades, the structure of a highly evolved complex multi-unit multi-enzyme complex, called the cellulosome, has been elucidated and it is known to be directly responsible for biomass carbohydrate hydrolysis. This enzyme complex, which can contain as many as 30 proteins with various functions, have been the subject of detailed structural biology research involving a number of clostridial species, including Clostridium thermoellum, C. cellulovorans and C. cellulolyticum, and the basic structure of he cellulosome is similar.These anaerobic cellulose-utilizing microorganisms are able to produce simultaneously this highly complex cellulosome, which requires a significant level of metabolic energy, while actively fermenting the resulting cellulose and hemicellulose degradation products to ethanol and other byproducts such as organic acids. The present author remained skeptical regarding the excessive energy requirement needed for enzyme production and sugar transport and fermentation in an energy-deficient anaerobic environment. However,
this changed when it was discovered that these microorganisms, such as C. thermocellum, preferentially produce and transport cellulose degradation units containing an average of four glucose units, thus conserving precious high-energy transport by obtaining three glucose molecules ‘free’ relative to phosphate-driven sugar transport.
The discovery of an explanation for the rapid anaerobic growth and fermentation capabilities of C. thermocellum elevated this and similar microorganisms to possible candidates for a viable industrial process for the production of ethanol or other selected fermentation products, including hydrogen. However, thus far candidate CBP microorganisms all lack the preferred characteristics for the ideal ethanologen, as shown in Table .2. Research efforts are under way in a number of laboratories to address many of these limitations to take advantage of the inherent biomass carbohydrate degradative capacity of these anaerobes.
Table .2 Preferred characteristics of an ideal CBP microorganism
3. Gasification for Ethanol
Thermochemical processing of biomass, as mentioned in the Introduction, is not the subject of this chapter, but it has the real advantage in that any carbon-containing material can be converted rapidly with no pretreatment. A process called gasification is a thermochemical conversion process conducted in the absence of oxygen. With this process, all carbon-based materials yield common molecular building blocks of CO2, CO, H2O and H2. Gasification with oxygen produces synthesis gas with typically about 40% CO, 40% H2, 3% CH4 and 17% CO2 on a volume basis. Typical uses include generation of heat and power or as feedstock for chemicals. However, the diversity of Nature has produced anaerobic microorganisms that are able to utilize the aforementioned gasified products to grow and produce byproducts of fermentation. One microorganism, C. ljungdahlii, is of particular interest because it produces primarily ethanol under certain conditions along with smaller amounts of acetic acid and hydrogen. Engineering research has been under way for over 15 years to develop a commercial process with this unique fermentation process45–48 and commercialization is apparently moving forward (http://alicoinc.com/news_releases/2007/03-01-07A.html). However, little genetic improvement has occurred with these types of microorganism, providing a potentially fertile ground for biotechnological improvement.
Biodiesel
Biodiesel is either a derivative of triglycerides resulting in a fatty acid methyl ester (FAME) or the result of thermochemical conversion of biomass. The feedstock for FAME biodiesel is typically soybean oil in the USA or rapeseed/canola in Europe or Canada, although waste grease and fat from food operations can serve as feedstocks. However, the conversion technology to produce the FAME is the very mature, low-cost chemical transesterification process, which permits little opportunity for alternative processes such as enzymatic esterification. The process entails blending a triglyceride with an alcohol, usually methanol, in molar excess with an alkaline catalyst such as methoxide that caused the methanol to replace glycerol ester to the fatty acid yielding free glycerol and a FAME. The most significant opportunity for process and product improvement lies in the selection of alternative crop species beyond soybeans, and canola fills this niche to some extent. However, the prime driver for alternative crop species is both high yield of oil per acre and an optimal degree of unsaturation. Soybeans typically yield about 376 lb per acre of oil whereas canola produces about twice that amount per acre.
The degree of unsaturation is important in order to provide a degree of cold tolerance when blended with petroleum diesel at the 20% level, but oxidative instability is caused by the excessive double bond content. Canola is nearly 92% unsaturated but 63% of the fatty acids have only one double bond compared with 84% and 25% for soybean oil, respectively. Ideal plant species, such as an improved canola that has higher productivity and is able to be grown at in warmer mid-latitudes, should be the subject of plant breeding and bioengineering. Recent
production expansion aims at producing 1.8 billion US gallons, but only 0.33 billion gallons are in production at present. Unfortunately, the current cost of soybean oil has risen from $0.31 to $0.55 per pound, causing plants to reconsider operation.Germany, the largest producer of biodiesel, is facing market problems for very different reasons as of January 2008 due to large Federal tax increases, and this threatens the otherwise huge production from canola/rapeseed.
الاكثر قراءة في مواضيع عامة في التقانة الإحيائية
اخر الاخبار
اخبار العتبة العباسية المقدسة
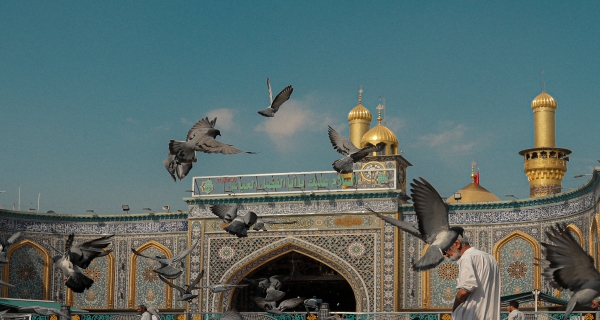
الآخبار الصحية
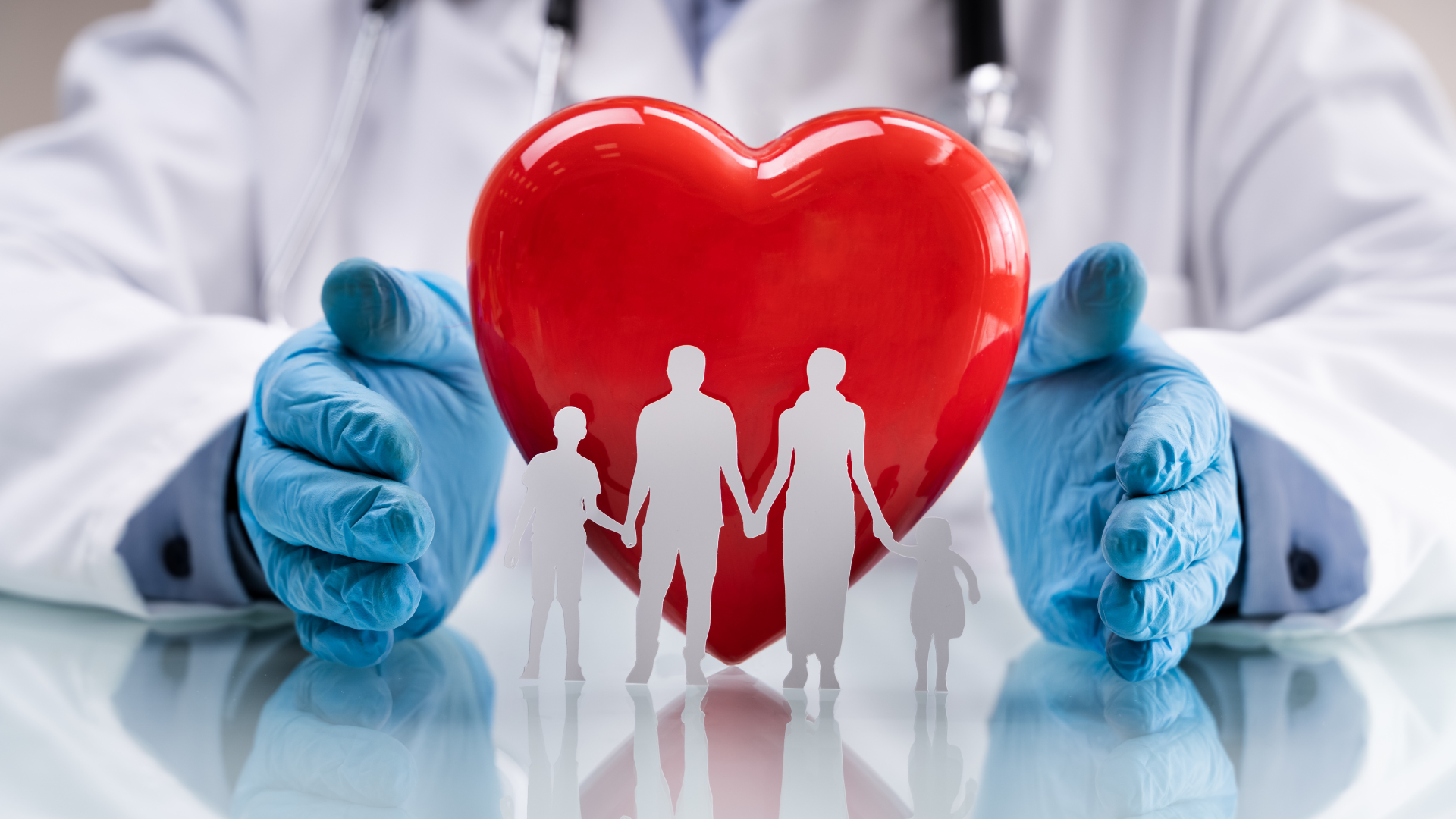