النبات
مواضيع عامة في علم النبات
الجذور - السيقان - الأوراق
النباتات الوعائية واللاوعائية
البذور (مغطاة البذور - عاريات البذور)
الطحالب
النباتات الطبية
الحيوان
مواضيع عامة في علم الحيوان
علم التشريح
التنوع الإحيائي
البايلوجيا الخلوية
الأحياء المجهرية
البكتيريا
الفطريات
الطفيليات
الفايروسات
علم الأمراض
الاورام
الامراض الوراثية
الامراض المناعية
الامراض المدارية
اضطرابات الدورة الدموية
مواضيع عامة في علم الامراض
الحشرات
التقانة الإحيائية
مواضيع عامة في التقانة الإحيائية
التقنية الحيوية المكروبية
التقنية الحيوية والميكروبات
الفعاليات الحيوية
وراثة الاحياء المجهرية
تصنيف الاحياء المجهرية
الاحياء المجهرية في الطبيعة
أيض الاجهاد
التقنية الحيوية والبيئة
التقنية الحيوية والطب
التقنية الحيوية والزراعة
التقنية الحيوية والصناعة
التقنية الحيوية والطاقة
البحار والطحالب الصغيرة
عزل البروتين
هندسة الجينات
التقنية الحياتية النانوية
مفاهيم التقنية الحيوية النانوية
التراكيب النانوية والمجاهر المستخدمة في رؤيتها
تصنيع وتخليق المواد النانوية
تطبيقات التقنية النانوية والحيوية النانوية
الرقائق والمتحسسات الحيوية
المصفوفات المجهرية وحاسوب الدنا
اللقاحات
البيئة والتلوث
علم الأجنة
اعضاء التكاثر وتشكل الاعراس
الاخصاب
التشطر
العصيبة وتشكل الجسيدات
تشكل اللواحق الجنينية
تكون المعيدة وظهور الطبقات الجنينية
مقدمة لعلم الاجنة
الأحياء الجزيئي
مواضيع عامة في الاحياء الجزيئي
علم وظائف الأعضاء
الغدد
مواضيع عامة في الغدد
الغدد الصم و هرموناتها
الجسم تحت السريري
الغدة النخامية
الغدة الكظرية
الغدة التناسلية
الغدة الدرقية والجار الدرقية
الغدة البنكرياسية
الغدة الصنوبرية
مواضيع عامة في علم وظائف الاعضاء
الخلية الحيوانية
الجهاز العصبي
أعضاء الحس
الجهاز العضلي
السوائل الجسمية
الجهاز الدوري والليمف
الجهاز التنفسي
الجهاز الهضمي
الجهاز البولي
المضادات الحيوية
مواضيع عامة في المضادات الحيوية
مضادات البكتيريا
مضادات الفطريات
مضادات الطفيليات
مضادات الفايروسات
علم الخلية
الوراثة
الأحياء العامة
المناعة
التحليلات المرضية
الكيمياء الحيوية
مواضيع متنوعة أخرى
الانزيمات
Related Codons Represent Chemically Similar Amino Acids
المؤلف:
JOCELYN E. KREBS, ELLIOTT S. GOLDSTEIN and STEPHEN T. KILPATRICK
المصدر:
LEWIN’S GENES XII
الجزء والصفحة:
29-5-2021
2217
Related Codons Represent Chemically Similar Amino Acids
KEY CONCEPTS
- Sixty-one of the 64 possible triplets together encode 20 amino acids.
- Three codons do not represent amino acids and cause termination of translation.
- The genetic code was established at an early stage of evolution and is nearly universal.
- Most amino acids are represented by more than one codon.
- The multiple codons for an amino acid are usually related.
- Chemically similar amino acids often have related codons, minimizing the effects of mutation.
The code is summarized in FIGURE 1. Because there are more codons than there are amino acids, the result is that almost all amino acids are represented by more than one codon. The only exceptions are methionine and tryptophan. Codons that encode the same amino acid are said to be synonymous. A polypeptide is actually translated from the mRNA, so the genetic code is usually described in terms of the four bases present in RNA: U, C, A, and G.
FIGURE .1 All the triplet codons have meaning: 61 represent amino acids and 3 cause termination (stop codons).
Codons representing the same or chemically similar amino acids tend to be similar in sequence. Often the base in the third position of a codon (its 3′ end) is not significant because the four codons differing only in the third base represent the same amino acid.
Sometimes a distinction is made only between a purine versus a pyrimidine in this position. The reduced specificity at the last position is known as third-base degeneracy.
To be interpreted, a codon in mRNA must first base pair with the anticodon of the corresponding aminoacyl-tRNA. This pairing occurs at the ribosome, where the interaction between complementary trinucleotides is stabilized by highly conserved 16S rRNA nucleotides in the A site. Strict monitoring of the overall basepair shape by rRNA permits only conventional A-U and G-C pairing to occur at the first two positions of the codon, but additional pairings are permitted at the third codon base, where rRNA contacts can follow different rules. As a result, a single aminoacylt RNAmay recognize more than one codon, by means of the additional, noncanonical pairs permitted at the third position.
Furthermore, pairing interactions may also be influenced by the posttranscriptional modification of tRNA, especially within or directly adjacent to the anticodon.
The tendency for identical or chemically similar amino acids to be represented by related codons minimizes the effects of mutations. It increases the probability that a single random base change will result in no amino acid substitution or in one involving amino acids of similar character. For example, a mutation of CUC to CUG does not change the resulting polypeptide because both codons represent leucine. Mutation of CUU to AUU results in replacement of leucine with isoleucine; both of these amino acids are hydrophobic and are likely to play similar roles in the encoded protein.
FIGURE .2 plots the number of codons representing each amino acid against the frequency with which the amino acid is used in proteins (in Escherichia coli). In general, amino acids that are more common are represented by more codons. This suggests that there has been some optimization of the genetic code with regard to the utilization of amino acids.
FIGURE .2 Some correlation of the frequency of amino acid use in proteins with the number of codons specifying the amino acid is observed. An exception is found for amino acids specified by two codons, which occur with a wide variety of frequencies.
The three codons (UAA, UAG, and UGA) that do not encode amino acids are used specifically to terminate translation. One of these stop codons marks the end of every open reading frame. Comparisons of DNA sequences with the corresponding polypeptide sequences reveal that an identical set of codon assignments is used in bacteria and in eukaryotes (except for some variations in mitochondria). As a result, mRNA from one species usually can be translated correctly in vitro or in vivo by the translation apparatus of another species. Thus, the codons used in the mRNA of one species have the same meaning for the ribosomes and tRNAs of other species.
The universality (with minor exceptions) of the genetic code suggests that it was established very early in evolution. Perhaps the code started in a primitive form in which a small number of codons were used to represent comparatively few amino acids, possibly even with one codon corresponding to any member of a group of amino acids. More precise codon meanings and additional amino acids could have been introduced later. One possibility is that at first only two of the three bases in each codon were used; discrimination at the third position could have evolved later.
Evolution of the code could have become “frozen” at a point at which the system had become so complex that any changes in codon meaning would disrupt functional proteins by substituting unacceptable amino acids. Its near universality implies that this must have happened at such an early stage that all living organisms are descended from a Last Universal Common Ancestor (LUCA) that used the current near-universal genetic code.
Exceptions to the universal genetic code are rare. Changes in meaning in the principal genome of a species usually concern the termination codons. For example, in a Mycoplasma, UGA encodes tryptophan; in certain species of the ciliates Tetrahymena and Paramecium UAA and UAG encode glutamine. Systematic alterations of the code have occurred only in mitochondrial DNA .
الاكثر قراءة في مواضيع عامة في الاحياء الجزيئي
اخر الاخبار
اخبار العتبة العباسية المقدسة
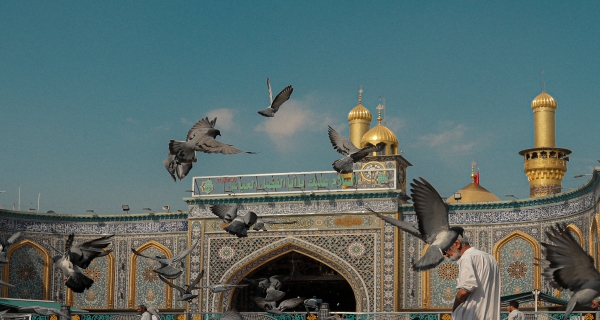
الآخبار الصحية
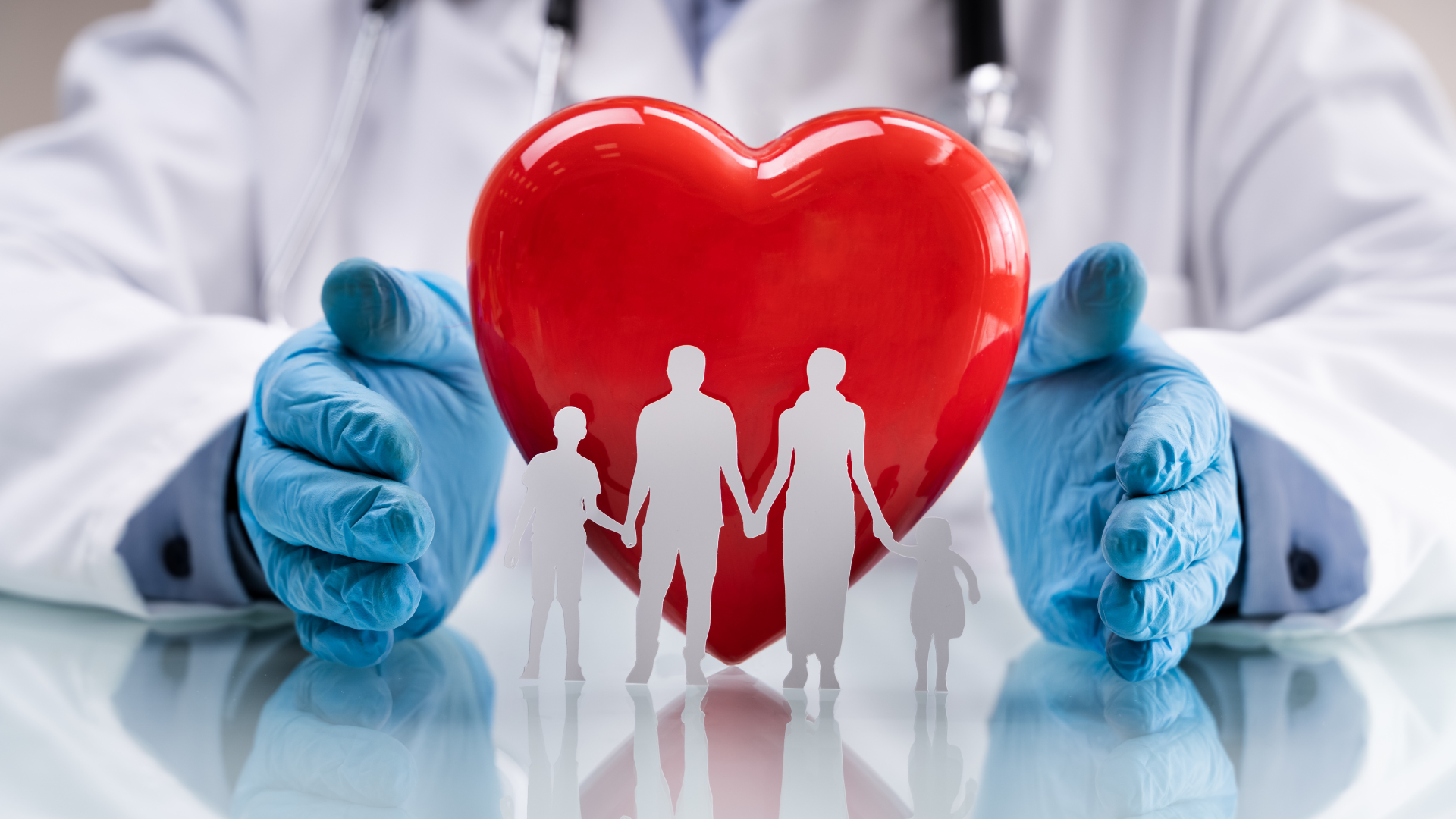