تاريخ الفيزياء
علماء الفيزياء
الفيزياء الكلاسيكية
الميكانيك
الديناميكا الحرارية
الكهربائية والمغناطيسية
الكهربائية
المغناطيسية
الكهرومغناطيسية
علم البصريات
تاريخ علم البصريات
الضوء
مواضيع عامة في علم البصريات
الصوت
الفيزياء الحديثة
النظرية النسبية
النظرية النسبية الخاصة
النظرية النسبية العامة
مواضيع عامة في النظرية النسبية
ميكانيكا الكم
الفيزياء الذرية
الفيزياء الجزيئية
الفيزياء النووية
مواضيع عامة في الفيزياء النووية
النشاط الاشعاعي
فيزياء الحالة الصلبة
الموصلات
أشباه الموصلات
العوازل
مواضيع عامة في الفيزياء الصلبة
فيزياء الجوامد
الليزر
أنواع الليزر
بعض تطبيقات الليزر
مواضيع عامة في الليزر
علم الفلك
تاريخ وعلماء علم الفلك
الثقوب السوداء
المجموعة الشمسية
الشمس
كوكب عطارد
كوكب الزهرة
كوكب الأرض
كوكب المريخ
كوكب المشتري
كوكب زحل
كوكب أورانوس
كوكب نبتون
كوكب بلوتو
القمر
كواكب ومواضيع اخرى
مواضيع عامة في علم الفلك
النجوم
البلازما
الألكترونيات
خواص المادة
الطاقة البديلة
الطاقة الشمسية
مواضيع عامة في الطاقة البديلة
المد والجزر
فيزياء الجسيمات
الفيزياء والعلوم الأخرى
الفيزياء الكيميائية
الفيزياء الرياضية
الفيزياء الحيوية
الفيزياء العامة
مواضيع عامة في الفيزياء
تجارب فيزيائية
مصطلحات وتعاريف فيزيائية
وحدات القياس الفيزيائية
طرائف الفيزياء
مواضيع اخرى
Ionization Energy
المؤلف:
Donald A. Neamen
المصدر:
Semiconductor Physics and Devices
الجزء والصفحة:
p 117
18-5-2017
1317
Ionization Energy
We can calculate the approximate distance of the donor electron from the donor impurity ion, and also the approximate energy required to elevate the donor electron into the conduction band. This energy is referred to as the ionization energy. We will use the Bohr model of the atom for these calculations. The justification for using this model is that the most probable distance of an electron from the nucleus in a hydrogen atom, determined from quantum mechanics, is the same as the Bohr radius. The energy levels in the hydrogen atom determined from quantum mechanics are also the same as obtained from the Bohr theory.
In the case of the donor impurity atom, we may visualize the donor electron orbiting the donor ion, which is embedded in the semiconductor material. We will need to use the permittivity of the semiconductor material in the calculations rather than the permittivity of free space as is used in the case of the hydrogen atom. We will all use the effective mass of the electron in the calculations.
The analysis begins by setting the coulomb force of attraction between the electron and ion equal to the centripetal force of the orbiting electron. This condition we give a steady orbit. We have
(1)
where v is the magnitude of the velocity and rn is the radius of the orbit. If we assort the angular momentum is also quantized, then we can write
(2)
where n is a positive integer. Solving for v from Equation (2), substituting in Equation (1), and solving for the radius, we obtain
(3)
The assumption of the angular momentum being quantized leads to the radius as being quantized.
The Bohr radius is defined as
(4)
We can normalize the radius of the donor orbital to that of the Bohr radius, which give
(5)
where ϵr is the relative dielectric constant of the semiconductor material, m0 is tires mass of an electron, and m* is the conductivity effective mass of and m* is the conductivity effective mass of the electron the electron the semiconductor.
If we consider the lowest energy state in which n = 1, and if we consider silos in which ϵr = 11.7 and the conductivity effective mass is m*/m0 = 0.26. then we have that
(6)
or r1 = 23.9 A. This radius corresponds to approximately four lattice constants silicon. Recall that one unit cell in silicon effectively contains eight atoms, so the radius of the orbiting donor electron encompasses many silicon atoms. The donor electron is not tightly bound to the donor atom.
The total energy of the orbiting electron is given by
(7)
where T is the kinetic energy and V is the potential energy of the electron. The kinetic energy is
(8)
Using the velocity v from Equation (2) and the radius rn from Equation (3), the kinetic energy becomes
(9)
The potential energy is
(10)
The total energy is the sum of the kinetic and potential energies, so that
(11)
For the hydrogen atom, m* = m0 and ϵ = ϵ0. The ionization energy of the hydrogen atom in the lowest energy state is then E = - 13.6 eV. If we consider silicon, the ionization energy is E = -25.8 m eV, much less than the bandgap energy of silicon. This energy is the approximate ionization energy of the donor atom, or the energy required to elevate the donor electron into the conduction band.
For ordinary donor impurities such as phosphorus or arsenic in silicon or germanium, this hydrogenic model works quite well and gives some indication of the magnitudes of the ionization energies involved. Table 1.1 lists the actual experimentally measured ionization energies for a few impurities in silicon and germanium. Germanium and silicon have different relative dielectric constants and effective masses; thus we expect the ionization energies to differ.
Table 1.1 Impurity ionization energies in silicon and germanium.
الاكثر قراءة في مواضيع عامة في الفيزياء الصلبة
اخر الاخبار
اخبار العتبة العباسية المقدسة
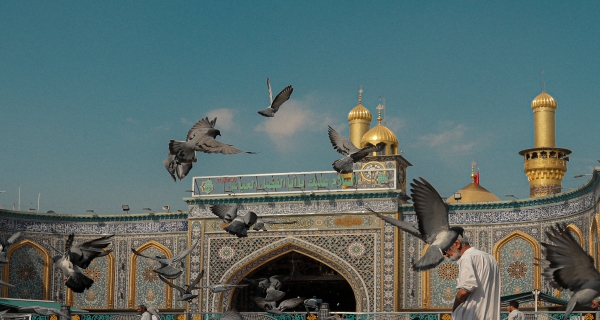
الآخبار الصحية
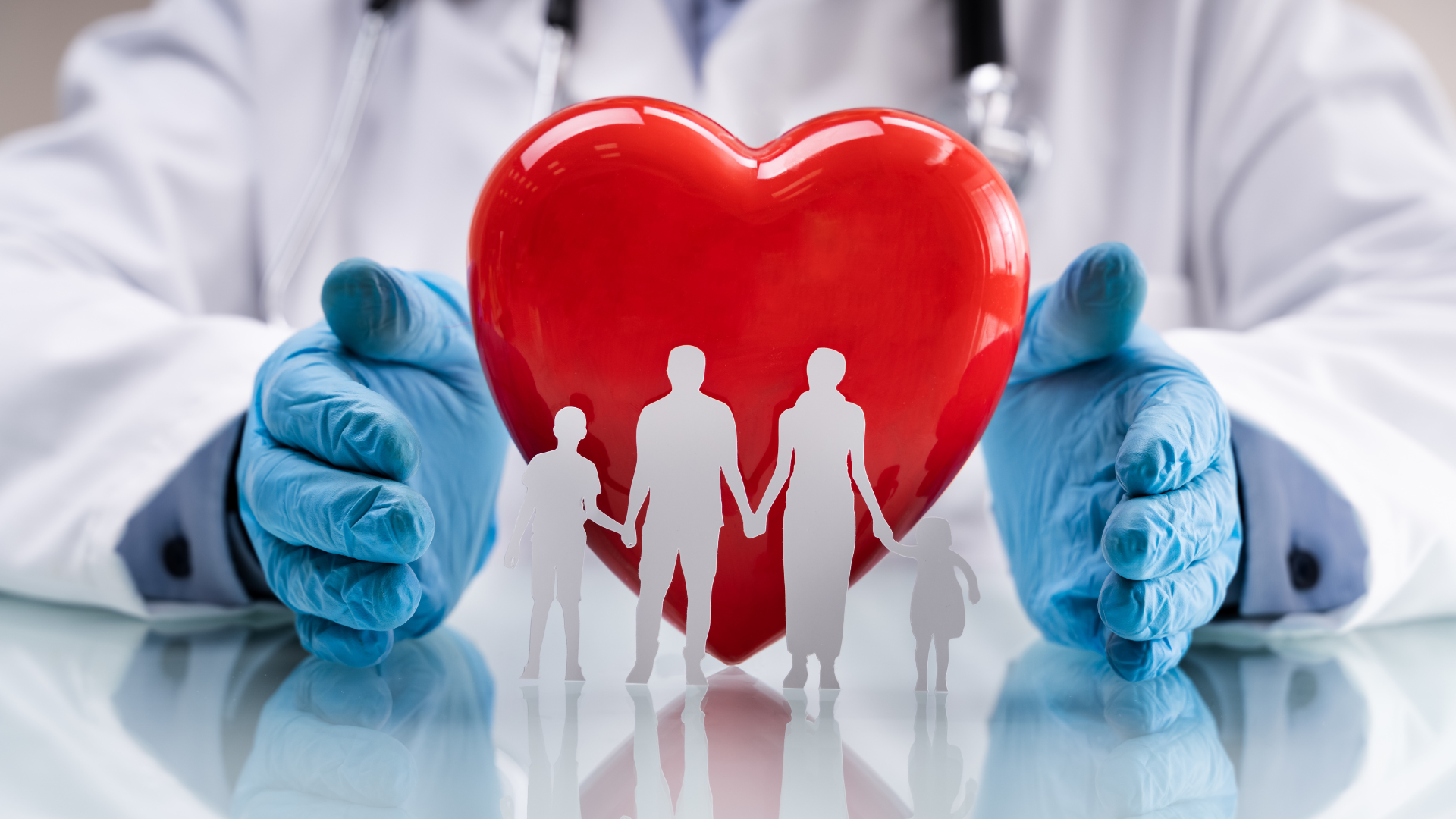