النبات
مواضيع عامة في علم النبات
الجذور - السيقان - الأوراق
النباتات الوعائية واللاوعائية
البذور (مغطاة البذور - عاريات البذور)
الطحالب
النباتات الطبية
الحيوان
مواضيع عامة في علم الحيوان
علم التشريح
التنوع الإحيائي
البايلوجيا الخلوية
الأحياء المجهرية
البكتيريا
الفطريات
الطفيليات
الفايروسات
علم الأمراض
الاورام
الامراض الوراثية
الامراض المناعية
الامراض المدارية
اضطرابات الدورة الدموية
مواضيع عامة في علم الامراض
الحشرات
التقانة الإحيائية
مواضيع عامة في التقانة الإحيائية
التقنية الحيوية المكروبية
التقنية الحيوية والميكروبات
الفعاليات الحيوية
وراثة الاحياء المجهرية
تصنيف الاحياء المجهرية
الاحياء المجهرية في الطبيعة
أيض الاجهاد
التقنية الحيوية والبيئة
التقنية الحيوية والطب
التقنية الحيوية والزراعة
التقنية الحيوية والصناعة
التقنية الحيوية والطاقة
البحار والطحالب الصغيرة
عزل البروتين
هندسة الجينات
التقنية الحياتية النانوية
مفاهيم التقنية الحيوية النانوية
التراكيب النانوية والمجاهر المستخدمة في رؤيتها
تصنيع وتخليق المواد النانوية
تطبيقات التقنية النانوية والحيوية النانوية
الرقائق والمتحسسات الحيوية
المصفوفات المجهرية وحاسوب الدنا
اللقاحات
البيئة والتلوث
علم الأجنة
اعضاء التكاثر وتشكل الاعراس
الاخصاب
التشطر
العصيبة وتشكل الجسيدات
تشكل اللواحق الجنينية
تكون المعيدة وظهور الطبقات الجنينية
مقدمة لعلم الاجنة
الأحياء الجزيئي
مواضيع عامة في الاحياء الجزيئي
علم وظائف الأعضاء
الغدد
مواضيع عامة في الغدد
الغدد الصم و هرموناتها
الجسم تحت السريري
الغدة النخامية
الغدة الكظرية
الغدة التناسلية
الغدة الدرقية والجار الدرقية
الغدة البنكرياسية
الغدة الصنوبرية
مواضيع عامة في علم وظائف الاعضاء
الخلية الحيوانية
الجهاز العصبي
أعضاء الحس
الجهاز العضلي
السوائل الجسمية
الجهاز الدوري والليمف
الجهاز التنفسي
الجهاز الهضمي
الجهاز البولي
المضادات الحيوية
مواضيع عامة في المضادات الحيوية
مضادات البكتيريا
مضادات الفطريات
مضادات الطفيليات
مضادات الفايروسات
علم الخلية
الوراثة
الأحياء العامة
المناعة
التحليلات المرضية
الكيمياء الحيوية
مواضيع متنوعة أخرى
الانزيمات
The T-Cell System
المؤلف:
T. Sargunam Stephen
المصدر:
Medical Microbiology
الجزء والصفحة:
8-11-2015
8687
The T-Cell System
T-Cell Receptors (TCR) and Accessory Molecules
Like B cells, T cells have receptors that bind specifically to their steric counterparts on antigen epitopes. The diversity of T-cell receptors is also achieved by means of genetic rearrangement of V, D, and J segments (Fig. 1b). However, the T-cell receptor is never secreted, and instead remains membrane-bound.
Each T-cell receptor consists of two transmembrane chains, of either the α and β forms, or the γ andδ forms (not to be confused with the heavy chains of Ig bearing the same designations). Both chains have two extracellular domains, a transmembrane anchor element and a short intracellular extension. As for Ig, the terminal domains are variable in nature (i.e., Va and Vb), and together they form the antigen binding site. T-cell receptors are associated with their so-called co-receptors—other membrane-enclosed proteins expressed on the T cell surface—which include the multiple-chain CD3 complex, and CD4 or CD8 molecules (depending on the specific differentiation of the T cell). CD stands for “cluster of differentiation” or “cluster determinant” and represents differentiation antigens defined by clusters of monoclonal antibodies.
Fig. 1 a Heavy chain of human IgG. The designations for the gene segments in the variable part of the H chain are V (variable), D (diversity), and J (joining). The segments designated as µ, δ, γ, α, and e code for the constant region and determine the immunoglobulin class. The V segment occurs in several hundred versions, the D segment in over a dozen, and the J segment in several forms. V, D, and J segments combine randomly to form a sequence (VDJ) which codes for the variable part of the H chain. This rearranged DNA is then transcribed, creating the primary RNA transcript. The non-coding intervening sequences (introns) are then spliced out, and the resulting mRNA is translated into the protein product. b a chain of mouse T-cell receptor. Various different V, D, and J gene segments (for b and 5), V and J gene segments (for a and y) are available for the T-cell receptor chains. The DNA loci for the 5 chain genes are located between those for the a chain.
T-Cell Specificity and the Major Histocompatibility Complex (MHC)
T-cell receptors are unable to recognize free antigens. Instead the T-cell receptor can only recognize its specific epitope once the antigen has been cleaved into shorter peptide fragments by the presenting cell. These fragments must then be embedded within a specific molecular groove and presented to the T-cell receptor (a process known as MHC-restricted T-cell recognition or MHC restriction). This “binding groove” is located on the MHC molecule. The MHC encodes for the powerful histocompatibility or transplantation antigens (also known in humans as HLA, human leukocyte antigen molecules, Fig. 2).
The designation “MHC molecule” derives from the initial discovery of the function of the complex as a cell surface structure, responsible for the
Fig. 2 The human major histocompatibility gene complex (HLA genes) is located on chromosome 6. There are three different classes of MHC molecules.
immunological rejection of cell transfusions or tissue and organ transplants. Its true function as a peptide-presenting molecule was not discovered until the seventies, when its role became apparent whilst testing the specificity of virus-specific cytotoxic T cells. During these experiments it was observed that immune T cells were only able to destroy infected target cells if both cell types were derived from the same patient or from mice with identical MHC molecules. The resulting conclusion was that a T-cell receptor not only recognizes the corresponding amino acid structure of the presented peptide, but additionally recognizes certain parts of the MHC structure. It is now known that this contact between MHC on the APC and the T-cell receptor is stabilized by the co-receptors CD4 and CD8.
MHC classes. Molecules encoded by the MHC can be classified into three groups according to their distribution on somatic cells, and the types of cells by which they are recognized:
- -MHC class I molecules. These molecules consist of a heavy a chain with three Ig-like polymorphic domains (these are encoded by 100-1000 alleles, with the a1 and a2 domains being much more polymorphic than the a3 domain) and a nonmembrane-bound (soluble) single-domain b2 microglobulin (b2M, which is encoded by a relatively small number of alleles). The a chain forms a groove that functions to present antigenic peptides (Fig. 3). Human HLA-A, HLA-B, and HLA-C molecules are expressed in varying densities on all somatic cells (the relative HLA densities for fibroblasts and hepatic cells, lymphocytes, or neurons are 1x, 100x and 0.1 x, respectively). Additional, non- classical, class I antigens which exhibit a low degree of polymorphism are also present on lymphohematopoietic cells and play a role in cellular differentiation.
- -MHC class II molecules. These are made up by two different polymorphic transmembrane chains that consist of two domains each (a1 is highly polymorphic, whilst b1 is moderately polymorphic, and b2 is fairly constant). These chains combine to form the antigen-presenting groove. Class II molecules are largely restricted to lymphohematopoetic cells, antigen-presenting cells (APC), macrophages, and so on. In humans, but not in mice, they are also found on some epithelial cells, neuroendocrine cells, and T cells. The products of the three human gene regions HLA-DP, HLA-DQ and HLA-DR can additionally form molecules representing combinations of two loci—thus providing additional diversity for peptide presentation.
-MHC class III molecules. These molecules are not MHC antigens in the classical sense, but are encoded within the MHC locus. These include complement (C) components C4 and C2, cytokines (IL, TNF), heat shock protein 70 (hsp70), and other products important for peptide presentation.
Fig. 3 MHC class I translation antigen: a lateral view, b vertical view. The presenting peptide is shown in violet. The three domains of the heavy chain are a1, a2 and a3. b2 microglobulin (b2M) functions as a light chain, and is not covalently bound to the heavy chain.
Functions of MHC molecules. MHC class I and II molecules function mainly as molecules capable of presenting peptides . These two classes of MHC molecules present two different types of antigens:
- -Intracellular antigens; these are cleaved into peptides within the proteasome and are usually associated with MHC class I molecules via the endogenous antigen processing pathway (Fig. 4, left side).
- -Antigens taken up from exogenous sources; these are processed into peptides within phagolysosomes, and in most cases are then presented on MHC class II molecules on the cell surface . Within the phagolysosome, a fragment called the invariant chain (CLIP, class II- inhibiting protein) is replaced by an antigen fragment. This CLIP fragment normally blocks the antigen-binding site of the MHC class II dimer, thus preventing its occupation by other intracellular peptides.
The presentation groove of MHC class I molecules is closed at both ends, and only accommodates peptides of roughly 8-10 (usually 9) amino acids in
Fig. 4 Intracellularly synthesized endogenous antigen peptides (left side) are bound to MHC class I molecules within the endoplasmic reticulum, fixed into the groove by b2M, and presented on the cell surface. Antigens taken up from exogenous sources (right) are cleaved into peptides within phagosomes. The phagosome then merges with endosomes containing MHC class II molecules, the binding site of which had been protected by the so-called CLIP fragment. These two presentation pathways functionally separate MHC class I restricted CD8+Tcells from MHC class II restricted CD4+ T cells.
length. The groove of MHC class II molecules is open-ended, and can contain peptides of 9-15 (usually 10-12) amino acids in length.
T cells can only recognize antigenic peptides in combination with either MHC class I (which presents endogenous linear peptides, such as those derived from viruses) or MHC class II (which present exogenous linear peptides, such as those derived from bacterial toxins) (Table 2.3). In contrast to antibodies that recognize soluble, complex, nonlinear, three-dimensional structures—T-cell recognition is restricted to changes on the surfaces of cells signaled via MHC plus peptide.
T-cell specificity. T-cell recognition therefore involves two levels of specificity: first, MHC presentation molecules bind peptides with a certain degree of specificity as determined by the shape of the groove and the peptide anchoring loci. Second, the MHC-peptide complex will only be recognized by specific T-cell receptors (TCR) once a minimum degree of binding strength has been obtained. For this reason diseases associated with the HLA complex are determined largely by the quality of peptide presentation, but can also be influenced by the available TCR repertoire.
The structure of the MHC groove therefore determines which, of all the potentially recognizable, peptides will actually be presented as T-cell epitopes. Thus, the same peptides cannot function as T-cell epitopes in all individuals. Nonetheless, certain combinations of peptides and MHC are frequently observed. For example, approximately 50% of Caucasians carry the HLA-A2 antigen, although this is sometimes found in a variant form.
Antigen-presenting cells (APC). APCs belong to the lymphohematopoietic system. They attach peptides to MHC class II molecules for presentation to T cells, and induce T-cell responses. The complex mechanisms involved in this process have not yet been fully delineated. Stromal cells present in the thymus and bone marrow (i.e., connective tissue cells, dendritic cells and nurse cells in both thymus and bone marrow, plus epithelial cells in the thymus) can also function as APCs. The following cell types function as APCs in peripheral secondary lymphoid organs:
-Circulating monocytes
Sessile macrophages in tissues, microglia in the central nervous system-
-Bone marrow derived dendritic cells with migratory potential—these occur as cutaneous Langerhans cells, as veiled cells during antigen transfer into the afferent lymph vessels, as interdigitating cells in the spleen and lymph nodes, and as interstitial dendritic cells or as M cells within MALT
-Follicular dendritic cells (FDC)—these are found within the germinal centers of the secondary lymph organs, do not originate in the bone marrow, and do not process antigens but rather bind antigen-antibody complexes via Fc receptors and complement (C3) receptors
-B lymphocytes—these serve as a type of APC for T helper cells during T-B collaborations.
The consequences of MHC variation. Because every individual differs with regard to the set of polymorphic MHC molecules and self antigens expressed (with the exception of monozygotic twins and inbred mice of the same strain), 2 the differences between two given individuals are considerable. The high degree of variability in MHC molecules—essential for the presentation of a large proportion of possible antigenic peptides for T-cell recognition—results in these molecules becoming targets for T cell recognition following cellular or organ transplantation resulting in transplant rejection. The term “transplantation antigens” is therefore a misnomer, and is only used because their real function was not discovered until a later time. Normally antigens are only recognized by T cells if they are associated with MHC-encoded self-structures. Transplant recognition, which apparently involves the imitation of the com-bination of a non-self antigen plus a self-MHC molecule, can therefore be considered an exception. The process probably arises from T-cell receptor crossreactivity between host self-MHC antigens plus foreign peptides on the one hand, and non-self transplantation antigens associated with self-peptides from the donor on the other hand (for example, the T-cell receptor for HLA-A2 peptide X cross-reacts with HLA-A13 peptide Y). Transplant rejection is therefore a consequence of the enormous variety of combinations of antigenic peptide plus MHC, which is exhibited by each individual organism.
Fig. 5 a The interactions of APCs or B cells with CD4+ T cells (T helper cells) are mediated by MHC class II molecules (heterodimers). b Interactions between CD8+ T cells (cytotoxic T cells) and their target cells are mediated by MHC class I molecules. The presenting peptide is shown in violet. ”S” indicates a superantigen, named after its capacity to activate many different T helper cells through its ability to bind to the constant regions of both the MHC and TCR molecules (naive = nonactivated T cells, act. = activated T cells).
T-Cell Maturation: Positive and Negative Selection
Maturation of Tcells occurs largely within the thymus. Because the MHC-encoded presentation molecules are highly polymorphic, and are also subject to mutation, the repertoire of TCRs is not genetically pre-determined. One prerequisite for an optimal repertoire of T-cells is therefore the positive selection of T cells such that these preferentially recognize peptides associated only with self transplantation (MHC) antigens. A second prerequisite is negative selection, which involves the deletion of T cells that react too strongly against self MHC plus self peptide. The random processes governing the genetic generation of an array of T-cell receptors results αβ or γδ receptor chain combinations which are in the majority of cases are non-functional. Those T cells preserved through to maturity represent cells carrying receptors capable of effectively recognizing self-MHC molecules (positive selection). However, the T cells within this group which express too high an affinity for self-MHC plus self-peptides are deleted (negative selection).
The process of positive selection was demonstrated in experimental mice expressing MHC class I molecules of type b (MHC classIb) from which the thymus had been removed (and which therefore had noT cells). Implantation of a new thymus with MHC class I molecules of type a (MHC class Ia) into the MHC class Ib mice resulted in the maturation of Tcells which only recognized peptides presented by MHC class Ia molecules, and not peptides presented by MHC class Ib molecules. However, recent experiments have shown that this is probable an experimental artefact and that it is not (or not solely) the thymic epithelial cells that determine the selection process, but that this process is driven by cells formed in the bone marrow. Positive selection is generally achieved by weak levels of binding affinity between the T-cell receptor and the self-MHC molecules, whereas negative selection eliminates those T cells exhibiting the highest levels of affinity (namely the self-or auto-reactive T cells) and absence of binding causes death by neglect. Thus, only T cells with moderate binding affinities are allowed to mature and exit the thymus. These T cells can potentially react to non-self (foreign) peptides presented by self MHC molecules. The enormous proliferation of immature thymocytes is paralleled by continuous cell death of large numbers of thymocytes. In general, the maturation and survival of lymphocytes is considered to be dependent on a continuous, repetitive, signaling via transmembrane molecules, and cessation of these signals is usually taken as a reliable indicator of cell death.
T-Cell Subpopulations
In order to recognize the presented antigen, T cells require the specific T-cell receptor and a molecule which functions to recognize the appropriate MHC molecules (i.e. CD4 or CD8 which recognize MHC class II and MHC class I, respectively). Thus T cells are classified into different subpopulations based on the CD4 or CD8 surface molecules:
CD4+ T cells. These T cells recognize only MHC class II-associated antigens. They are also called T helper cells due to their important role in T-B cell collaboration (Fig. 5a), although they exhibit many other additional functions. CD4+ cells can produce, or induce, the production of cytokines by which means they can activate macrophages and exercise a regulatory effect on other lymphocytes. Although these cells sometimes demonstrate an ability to cause cytotoxic destruction in vitro, this does not hold true in vivo.
CD8+ T cells. Only MHC class I-associated antigens are recognized by the CD8+ molecule. These cells are also known as cytotoxic T cells due to their ability to destroy histocompatible virus-infected, or otherwise altered, target cells as well as allogeneic cells. This effect can be observed both in vitro and in vivo (Fig. 5b). Costimulatory molecules are not required for this lyti effector function. However, cytotoxicity is only one of several important functions expressed by CD8+ T cells. They also have many other non-lytic functions which they execute via the production, or induction of, cytokine release. The designation (CD8+) T suppressor cell is misleading and should not be used. It was originally coined to distinguish these cells from the function of T helper cells, mentioned above. However, plausible documentation of a suppressor effect by CD8+ T cells has only been obtained in a very small number of cases. In most cases, this suppressive effect can in fact be explained by the direct elimination of APC (i.e., by changing the antigen kinetics), or indirectly via cytokine effects. Thus, the name suppressor T cell suggests a regulatory function that in reality is unlikely to exist. In general, more neutral names, such as CD4+ T cells or CD8+ T cells, are preferable. Whereas the cytotoxic effector cells in the spleen and lymph nodes possess a heterodimeric (α + β chain) CD8+ T molecule, the function of CD8+ T cells found in the intestinal wall and expressing the a-homodimeric CD8 molecule remains unclear.
γ T cells. As for the homologous αβ heterodimer, the γ δ T-cell receptor is associated with the CD3 complex within the cell membrane. The genetic sequence for the γ and δ T-cell receptor is associated with the CD3 complex within the cell membrane. The genetic sequence for the γ and δ chains resembles that of the α and β chains, however, there are a few notable differences. The gene complex encoding the δ chain is located entirely within the V and J segments of the α chain complex. As a result, any rearrangement of the α chain genes deletes the δ chain genes. There are also far fewer V segments for the y and § genes than for the α and β chains. It is possible that the increased binding variability of the δ chains makes up for the small number of V segments, as a result nearly the entire variability potential of the γδ receptor is concentrated within the binding region (Table 1). The amino acids coded within this region are presumed to form the center of the binding site.
T cells with y§ receptors recognize certain class I-like gene products in association with phospholipids and phosphoglycolipids. In peripheral lymphoid tissues, only a small number of T cells express the y§ and CD3 co-receptor, however, many of the T cells found within the mucosa and submucosa express y§ receptors.
γδT cells can be negative for CD4+ and CD8+, or express two a chains (but no b chain) of the CD8+ molecule. Although it is assumed that y§ T cells may be responsible for early, low-specificity, immune defense at the skin and mucosa, their specificities and effector functions are still largely unknown.
Table 1 Organization of the Genetic Regions for the Human Immunoglobulins and T-Cell Receptor s (TCR)
References
Zinkernagel, R. M. (2005). Medical Microbiology. Thieme.
الاكثر قراءة في المناعة
اخر الاخبار
اخبار العتبة العباسية المقدسة
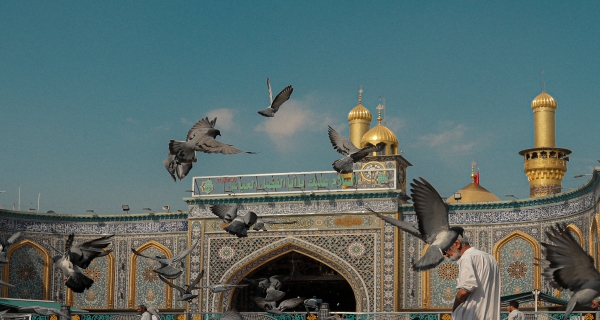
الآخبار الصحية
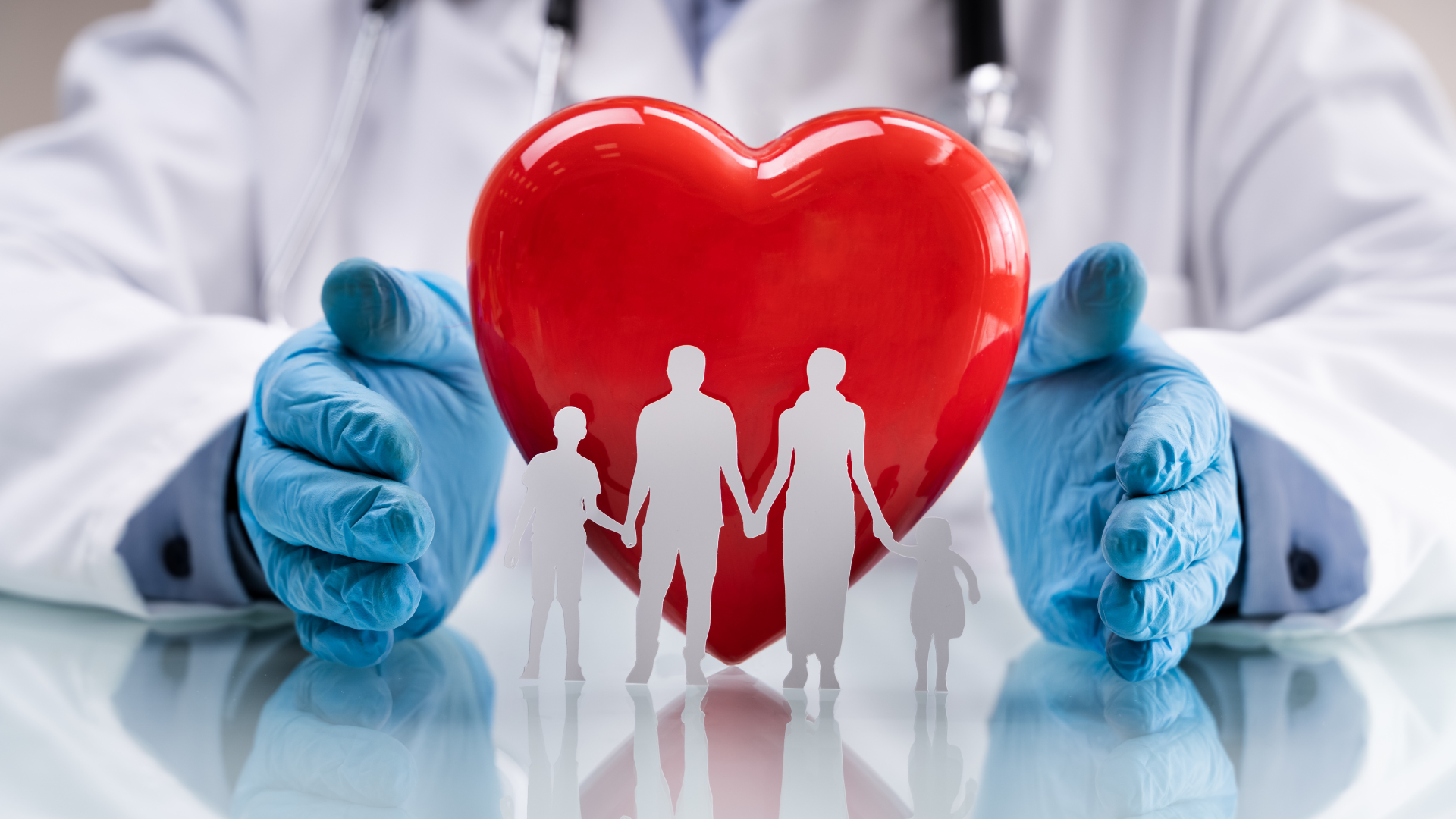