تاريخ الفيزياء
علماء الفيزياء
الفيزياء الكلاسيكية
الميكانيك
الديناميكا الحرارية
الكهربائية والمغناطيسية
الكهربائية
المغناطيسية
الكهرومغناطيسية
علم البصريات
تاريخ علم البصريات
الضوء
مواضيع عامة في علم البصريات
الصوت
الفيزياء الحديثة
النظرية النسبية
النظرية النسبية الخاصة
النظرية النسبية العامة
مواضيع عامة في النظرية النسبية
ميكانيكا الكم
الفيزياء الذرية
الفيزياء الجزيئية
الفيزياء النووية
مواضيع عامة في الفيزياء النووية
النشاط الاشعاعي
فيزياء الحالة الصلبة
الموصلات
أشباه الموصلات
العوازل
مواضيع عامة في الفيزياء الصلبة
فيزياء الجوامد
الليزر
أنواع الليزر
بعض تطبيقات الليزر
مواضيع عامة في الليزر
علم الفلك
تاريخ وعلماء علم الفلك
الثقوب السوداء
المجموعة الشمسية
الشمس
كوكب عطارد
كوكب الزهرة
كوكب الأرض
كوكب المريخ
كوكب المشتري
كوكب زحل
كوكب أورانوس
كوكب نبتون
كوكب بلوتو
القمر
كواكب ومواضيع اخرى
مواضيع عامة في علم الفلك
النجوم
البلازما
الألكترونيات
خواص المادة
الطاقة البديلة
الطاقة الشمسية
مواضيع عامة في الطاقة البديلة
المد والجزر
فيزياء الجسيمات
الفيزياء والعلوم الأخرى
الفيزياء الكيميائية
الفيزياء الرياضية
الفيزياء الحيوية
الفيزياء العامة
مواضيع عامة في الفيزياء
تجارب فيزيائية
مصطلحات وتعاريف فيزيائية
وحدات القياس الفيزيائية
طرائف الفيزياء
مواضيع اخرى
Electromagnetic Radiation
المؤلف:
Roger J Blin-Stoyle, FRS
المصدر:
Physics of Particles, Matter and the Universe
الجزء والصفحة:
p 56
22-5-2016
2546
Electromagnetic Radiation
In the preceding paragraphs we have seen that a steady current produces a magnetic field in its vicinity. Further, if the current is alternating i.e. electrons in the wire are oscillating then the associated magnetic field will also be continually changing-we have an oscillating magnetic field. However, as we have seen a changing magnetic field causes a current to flow in a wire; it must, therefore, have associated with it an electric field which causes the electrons to move along the wire. These two fields magnetic and electric will, of course, be present whether or not the wire, in which the electrons are being forced to move, is there. So we have the situation that oscillating electrons produce a combination of oscillating electric and magnetic fields which propagate out into space. This phenomenon is known as electromagnetic radiation and, at a fundamental level, is produced by accelerating electric charges, i.e. charges which are continually changing their motion. Bearing in mind the directions of the electric and magnetic forces indicated it is, perhaps, not surprising that the magnetic and electric fields are perpendicular to each other and also perpendicular to the direction in which they are being propagated (see figure 1.2(a)). When the alternating current is first switched on the propagation of the radiation is not instantaneous; it takes time for it to reach any point in space. It is rather analogous to the generation of a wave in a pond when a hand starts to wiggle the water at one side and a wave is propagated over the surface. The analogy is more than trivial because electromagnetic radiation is, indeed, a wave motion. However, there is a fundamental difference. With a water wave or a sound wave, the quantity that varies (water height, density of the air) is a property of the medium on or through which the wave is passing. However, with an electromagnetic wave, the varying elements are the electric and magnetic fields which are intrinsic to the wave itself and, as mentioned earlier, can be regarded as a ‘stress’ in space. This means that an electromagnetic wave can pass through a vacuum-it has no need of a medium in which to be propagated. The preceding discussion has been qualitative and it is gratifying that the relationship beween electricity, magnetism and electromagnetic radiation can be formulated in most elegant quantitative way; this was first done in the 1860s by a remarkable Scottish physicist James Clerk Maxwell. An electromagnetic wave can thus be represented as in figure 1.2(b) where the amplitude of the wave
Figure 1.2: (a) Electric and magnetic fields in electromagnetic radiation; (b) an electromagnetic wave.
measures the strength of the electric or magnetic field at a point in space. Since the electric and magnetic fields are perpendicular to the direction of travel, electromagnetic waves (like water waves) are said to be transverse. They move through empty space at a speed (always denoted by c) given by c = 2.998×108ms-1 or, approximately, 186,000 miles per second. Like mechanical waves they also carry energy with them whose value depends on the strengths of the electric and magnetic fields. Their nature depends on their frequency and wavelength which are simply related to each other and the speed of the wave. Examples of electromagnetic waves are visible light, ultraviolet and infrared rays, radio and television waves, x-rays etc. A rough indication of the wavelengths and frequencies of these different types of wave is given in table 1.1.
Table 1.1: Wavelengths and frequencies of different types of electromagnetic radiation.
There is clearly a vast spread in wavelengths and frequencies and the means of generation of these different waves varies. Radio, TV and radar waves are produced using complicated electronic devices. Infrared, visible, ultraviolet and x-rays are, as we shall see later, produced using atomic processes involving transitions of electrons mainly within atoms. Even higher frequency radiations, known as gamma rays, are produced when transitions take place within atomic nuclei themselves. That being said, and in spite of the great difference in the qualities of these different radiations, it must be stressed that the waves all have the same physical nature and the differences relate solely to the differences in their wavelengths and frequencies. Perhaps the most fully studied of all the electromagnetic radiations is visible light and we now go on to consider some aspects of its nature and behaviour.
الاكثر قراءة في الكهرومغناطيسية
اخر الاخبار
اخبار العتبة العباسية المقدسة
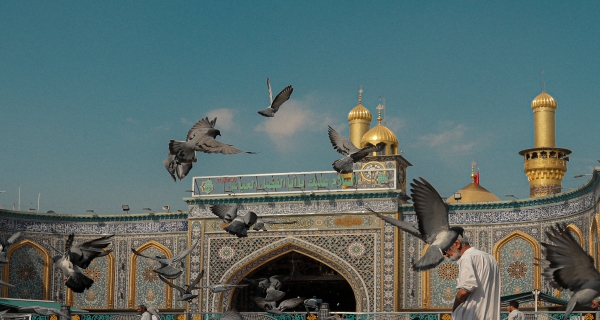
الآخبار الصحية
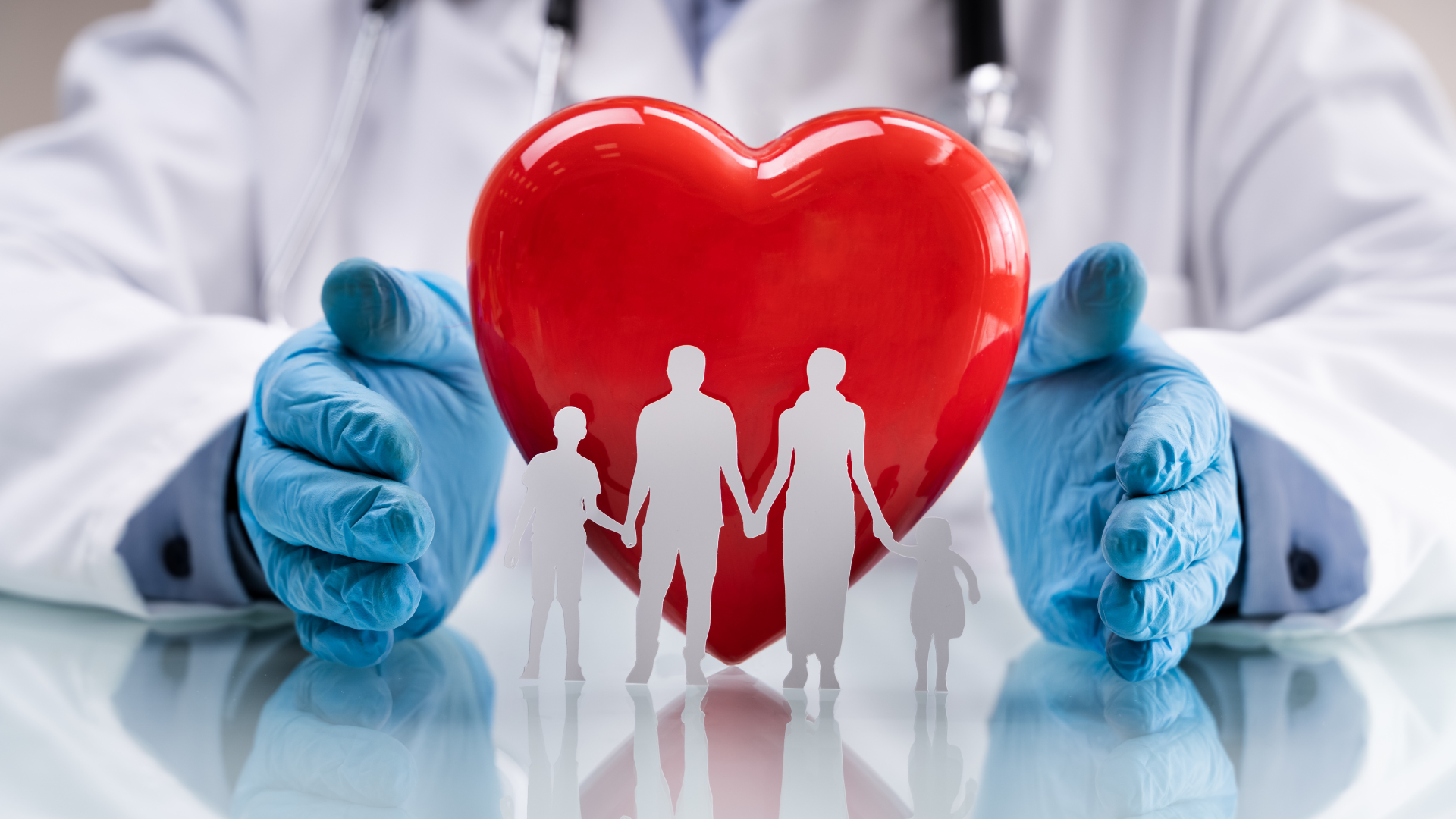