النبات
مواضيع عامة في علم النبات
الجذور - السيقان - الأوراق
النباتات الوعائية واللاوعائية
البذور (مغطاة البذور - عاريات البذور)
الطحالب
النباتات الطبية
الحيوان
مواضيع عامة في علم الحيوان
علم التشريح
التنوع الإحيائي
البايلوجيا الخلوية
الأحياء المجهرية
البكتيريا
الفطريات
الطفيليات
الفايروسات
علم الأمراض
الاورام
الامراض الوراثية
الامراض المناعية
الامراض المدارية
اضطرابات الدورة الدموية
مواضيع عامة في علم الامراض
الحشرات
التقانة الإحيائية
مواضيع عامة في التقانة الإحيائية
التقنية الحيوية المكروبية
التقنية الحيوية والميكروبات
الفعاليات الحيوية
وراثة الاحياء المجهرية
تصنيف الاحياء المجهرية
الاحياء المجهرية في الطبيعة
أيض الاجهاد
التقنية الحيوية والبيئة
التقنية الحيوية والطب
التقنية الحيوية والزراعة
التقنية الحيوية والصناعة
التقنية الحيوية والطاقة
البحار والطحالب الصغيرة
عزل البروتين
هندسة الجينات
التقنية الحياتية النانوية
مفاهيم التقنية الحيوية النانوية
التراكيب النانوية والمجاهر المستخدمة في رؤيتها
تصنيع وتخليق المواد النانوية
تطبيقات التقنية النانوية والحيوية النانوية
الرقائق والمتحسسات الحيوية
المصفوفات المجهرية وحاسوب الدنا
اللقاحات
البيئة والتلوث
علم الأجنة
اعضاء التكاثر وتشكل الاعراس
الاخصاب
التشطر
العصيبة وتشكل الجسيدات
تشكل اللواحق الجنينية
تكون المعيدة وظهور الطبقات الجنينية
مقدمة لعلم الاجنة
الأحياء الجزيئي
مواضيع عامة في الاحياء الجزيئي
علم وظائف الأعضاء
الغدد
مواضيع عامة في الغدد
الغدد الصم و هرموناتها
الجسم تحت السريري
الغدة النخامية
الغدة الكظرية
الغدة التناسلية
الغدة الدرقية والجار الدرقية
الغدة البنكرياسية
الغدة الصنوبرية
مواضيع عامة في علم وظائف الاعضاء
الخلية الحيوانية
الجهاز العصبي
أعضاء الحس
الجهاز العضلي
السوائل الجسمية
الجهاز الدوري والليمف
الجهاز التنفسي
الجهاز الهضمي
الجهاز البولي
المضادات الحيوية
مواضيع عامة في المضادات الحيوية
مضادات البكتيريا
مضادات الفطريات
مضادات الطفيليات
مضادات الفايروسات
علم الخلية
الوراثة
الأحياء العامة
المناعة
التحليلات المرضية
الكيمياء الحيوية
مواضيع متنوعة أخرى
الانزيمات
lac Repressor Binding to the Operator Is Regulated by an Allosteric Change in Conformation
المؤلف:
JOCELYN E. KREBS, ELLIOTT S. GOLDSTEIN and STEPHEN T. KILPATRICK
المصدر:
LEWIN’S GENES XII
الجزء والصفحة:
3-6-2021
2510
lac Repressor Binding to the Operator Is Regulated by an Allosteric Change in Conformation
KEY CONCEPTS
- The lac repressor protein binds to the double-stranded DNA sequence of the operator.
- The operator is a palindromic sequence of 26 bp.
- Each inverted repeat of the operator binds to the DNAbinding site of one repressor subunit.
- Binding of the inducer causes a change in the conformation of the repressor that reduces its affinity for DNA and releases it from the operator.
How does the repressor recognize the specific sequence of operator DNA? The operator has a feature common to many recognition sites for regulator proteins: It is a type of palindrome known as an inverted repeat. The inverted repeats are highlighted in FIGURE 1. Each repeat can be regarded as a half-site of the operator. The symmetry of the operator matches the symmetry of the repressor protein dimer. Each DNA-binding domain of the identical subunits in a repressor can bind one half-site of the operator; two DNA-binding domains of a dimer are required to bind the full-length operator. FIGURE 2 shows that the two DNAbinding domains in a dimeric unit contact DNA by inserting into successive turns of the major groove. This enormously increases affinity for the operator. Note that the lac operator is not a perfectly symmetrical sequence; it contains a single central base pair, and the sequence of the left side binds to the repressor more strongly than the sequence of the right side. An artificial, perfectly palindromic operator sequence binds to the lac repressor protein 10 times more tightly than the natural sequence!
FIGURE 1 The lac operator has a symmetrical sequence. The sequence is numbered relative to the start point for transcription at +1. The pink arrows to the left and to the right identify the two dyad repeats. The green blocks indicate the positions of identity.
FIGURE 2. The inducer changes the structure of the core so that the headpieces of a repressor dimer are no longer in an orientation with high affinity for the operator.
The importance of particular bases within the operator sequence can be determined by identifying those that contact the repressor protein or in which mutations change the binding of repressor. The lac repressor dimer contacts the operator in such a way that each inverted repeat of the operator makes the same pattern of contacts with a repressor monomer. This is shown by symmetry in the contacts that the repressor makes with the operator (the pattern between +1 and +6 is identical to that between +21 and +16) and by matching constitutive mutations in each inverted repeat, as shown in FIGURE 3. The region of DNA contacted by protein extends for 26 bp, and within this region are eight sites at which constitutive mutations occur. This emphasizes the same point made by promoter mutations: A small number of essential specific contacts within a larger region can be responsible for sequence-specific association of a protein binding to DNA.
FIGURE 3. Bases that contact the repressor can be identified by chemical crosslinking or by experiments to see whether modifications prevent binding. They identify positions on both strands of DNA extending from +1 to +23. Constitutive mutations occur at eight positions in the operator between +5 and +17.
Figure 2. shows another key element of repressor–operator binding: the insertion of the hinge helix into the minor groove of operator DNA, which bends the DNA by approximately 45°. This bend orients the major groove for HTH binding. DNA bending is commonly seen when a sequence is bound to a regulatory protein, illustrating the principle that the structure of DNA is more complicated than the canonical double helix.
The interaction between the lac repressor protein and the operator DNA is altered when the repressor is induced as shown in FIGURE 4 . Binding of the inducer (e.g., allolactose or IPTG) causes an immediate conformational change in the repressor protein. The change probably disrupts the hinge helices, changing the orientation of the headpieces relative to the core, with the result that the repressor’s affinity for DNA is lowered dramatically. Although the repressor has weak affinity for operator DNA, other sequences of genomic DNA can bind to the repressor with similar affinity. Thus, the operator and other DNA are in competition for the repressor protein. A cell contains much more genomic DNA than the single copy of the operator sequence; as a result, the genomic DNA “wins” the repressor protein, and the operator is vacant.
FIGURE 4 Does the inducer bind to the free repressor to upset an equilibrium (left) or directly to the repressor bound at the operator (right)?
Some structural and molecular details of the induction process remain the subject of active research. The number of inducers that must be bound to a dimer (within the tetramer) in order to cause induction is under debate. The nature of the conformational change caused in lac repressor by binding to inducer is also not completely known, because no high-resolution structure has been obtained for the repressor–operator–inducer complex. In the absence of DNA, inducer binding causes a change in the orientation of the core subdomains that are closest to the hinge helices. A similar change might occur when inducer binds to the repressor–operator complex. Such a change could disrupt the relative orientations of the hinge helices, lowering affinity for DNA. Low-resolution structural information of the low-affinity repressor–operator–inducer complex shows that the conformational changes in the induced lac repressor are probably not very large.
الاكثر قراءة في مواضيع عامة في الاحياء الجزيئي
اخر الاخبار
اخبار العتبة العباسية المقدسة
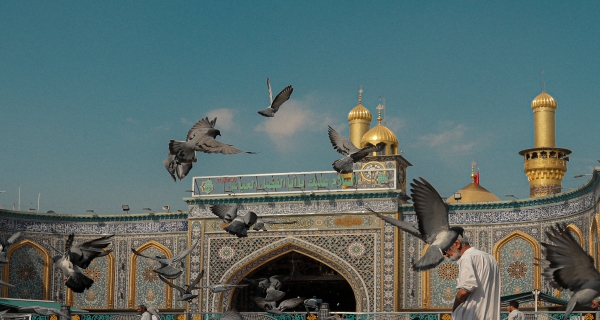
الآخبار الصحية
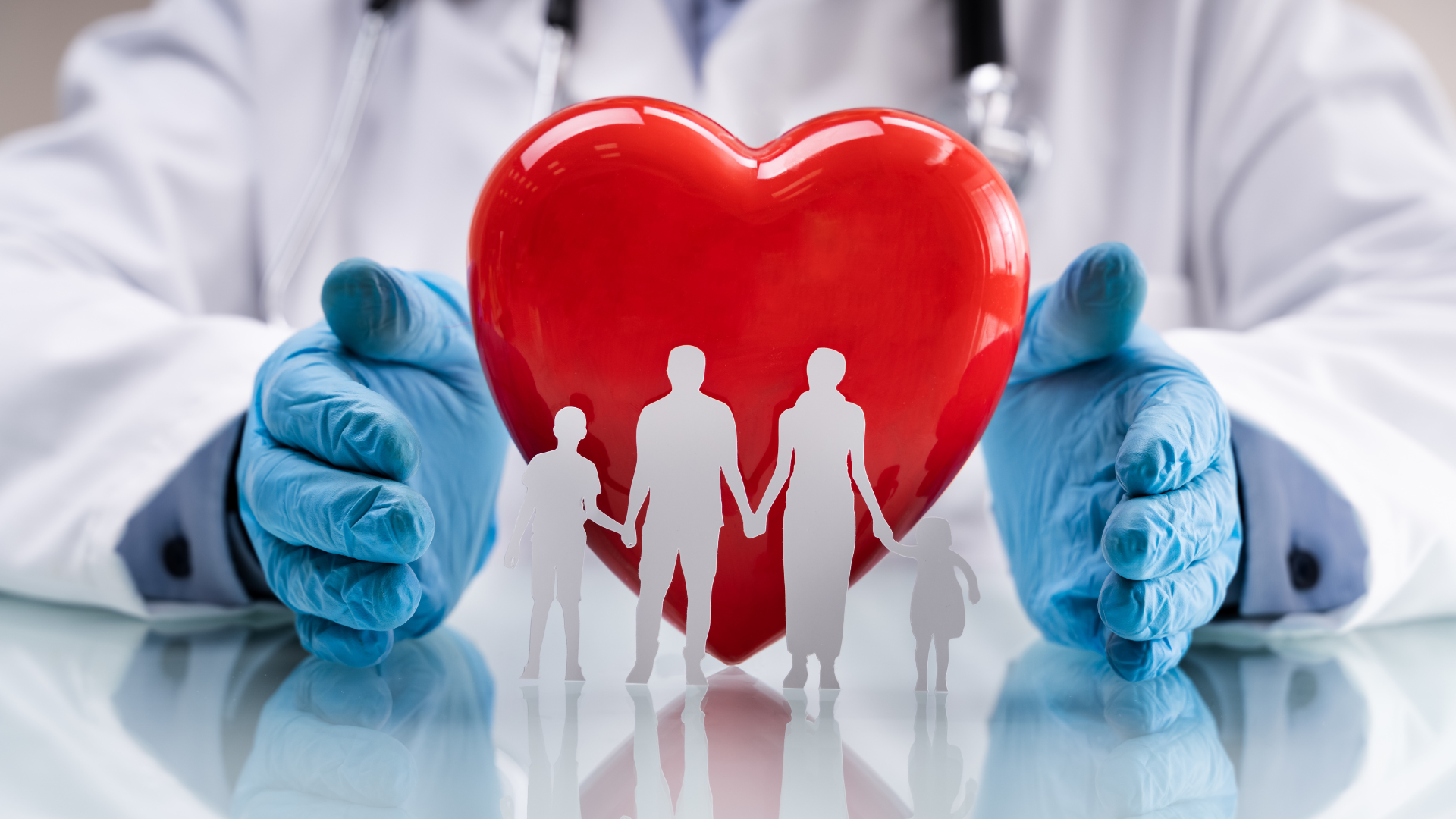