النبات
مواضيع عامة في علم النبات
الجذور - السيقان - الأوراق
النباتات الوعائية واللاوعائية
البذور (مغطاة البذور - عاريات البذور)
الطحالب
النباتات الطبية
الحيوان
مواضيع عامة في علم الحيوان
علم التشريح
التنوع الإحيائي
البايلوجيا الخلوية
الأحياء المجهرية
البكتيريا
الفطريات
الطفيليات
الفايروسات
علم الأمراض
الاورام
الامراض الوراثية
الامراض المناعية
الامراض المدارية
اضطرابات الدورة الدموية
مواضيع عامة في علم الامراض
الحشرات
التقانة الإحيائية
مواضيع عامة في التقانة الإحيائية
التقنية الحيوية المكروبية
التقنية الحيوية والميكروبات
الفعاليات الحيوية
وراثة الاحياء المجهرية
تصنيف الاحياء المجهرية
الاحياء المجهرية في الطبيعة
أيض الاجهاد
التقنية الحيوية والبيئة
التقنية الحيوية والطب
التقنية الحيوية والزراعة
التقنية الحيوية والصناعة
التقنية الحيوية والطاقة
البحار والطحالب الصغيرة
عزل البروتين
هندسة الجينات
التقنية الحياتية النانوية
مفاهيم التقنية الحيوية النانوية
التراكيب النانوية والمجاهر المستخدمة في رؤيتها
تصنيع وتخليق المواد النانوية
تطبيقات التقنية النانوية والحيوية النانوية
الرقائق والمتحسسات الحيوية
المصفوفات المجهرية وحاسوب الدنا
اللقاحات
البيئة والتلوث
علم الأجنة
اعضاء التكاثر وتشكل الاعراس
الاخصاب
التشطر
العصيبة وتشكل الجسيدات
تشكل اللواحق الجنينية
تكون المعيدة وظهور الطبقات الجنينية
مقدمة لعلم الاجنة
الأحياء الجزيئي
مواضيع عامة في الاحياء الجزيئي
علم وظائف الأعضاء
الغدد
مواضيع عامة في الغدد
الغدد الصم و هرموناتها
الجسم تحت السريري
الغدة النخامية
الغدة الكظرية
الغدة التناسلية
الغدة الدرقية والجار الدرقية
الغدة البنكرياسية
الغدة الصنوبرية
مواضيع عامة في علم وظائف الاعضاء
الخلية الحيوانية
الجهاز العصبي
أعضاء الحس
الجهاز العضلي
السوائل الجسمية
الجهاز الدوري والليمف
الجهاز التنفسي
الجهاز الهضمي
الجهاز البولي
المضادات الحيوية
مواضيع عامة في المضادات الحيوية
مضادات البكتيريا
مضادات الفطريات
مضادات الطفيليات
مضادات الفايروسات
علم الخلية
الوراثة
الأحياء العامة
المناعة
التحليلات المرضية
الكيمياء الحيوية
مواضيع متنوعة أخرى
الانزيمات
Cyclic GMP
المؤلف:
N. B. Bhatnagar, R. Bhatnagar, and T. A. Venkitasubramanian
المصدر:
Biochem. Biophys. Res. Commun., 121, 634–640
الجزء والصفحة:
29-12-2015
2774
Cyclic GMP (Cyclic Guanosine 3′,5′-Monophosphate, cGMP(
Cyclic GMP, a structural analog of cyclic AMP, occurs at similarly low concentrations (i.e., in the micromolar range) in many animal tissues. It is synthesized by guanylate cyclases, which correspond to the adenylate cyclases that synthesize cyclic AMP, and is destroyed by specific phosphodiesterases.
A functional role for cyclic GMP in bacteria is controversial. It is present in Escherichia coli but at such a low intracellular concentration (nanomolar, corresponding to about one molecule per cell) that hardly makes it a significant molecule. In addition, the sequence of the E. coli genome does not reveal any gene product that could code for a guanylate cyclase. There are many reports suggesting the presence of cGMP in bacteria (1), but its presence is perhaps most likely in myxobacteria, where it could be involved in cell aggregation and differentiation.
In contrast, cGMP is universally in eukaryotes (except in plants). It is generally involved in processes leading to activation of specific regulation cascades, which are differentially controlled by appropriate mediators, or leading to control specific processes for neuronal activation of sense organs, such as the sensitivity to light of retina receptor cells and the triggering of olfaction and taste (2-4) . The organization of guanylate cyclase and the control elements is sometimes similar to but distinct from the organization of hormonally regulated adenylate cyclase. In particular, G-protein-mediated regulation of vision operates on the phosphodiesterase rather than on the cyclase.
Phototransduction systems in vertebrates and invertebrates share a great deal of overall strategic similarity but differ significantly in the underlying molecular machinery. In the dark, vertebrate retinal rod cells synthesize a high level of cGMP that keeps gated sodium channels open in the plasma membrane of the outer segment (5). Light closes these channels by activating an enzymatic cascade that leads to rapid hydrolysis of cGMP by cGMP-specific phosphodiesterase (6). This hyperpolarizes the cell and modulates transmitter release at the synaptic buttons. Photoexcited rhodopsin triggers a transducer protein (transducin), which is related to G-proteins by catalyzing the exchange of GTP for bound GDP. Subsequently, the activated GTP-form of transducin switches on the phosphodiesterase. The cascade that has an overall gain of 105, is turned off by the GTPase activity of transducin and by the action of two proteins, rhodopsin kinase and arrestin . The kinetics of the reactions in the cGMP cascade limit the temporal resolution of the visual system, and statistical fluctuations in the reactions limit the reliability of detecting dim light. Together with calcium ions and inositol phosphates, cGMP controls visual excitation and adaptation. A light-induced fall in the internal free Ca2+ concentration subsequently stimulates resynthesis of cGMP, antagonizes the catalytic activity of rhodopsin, and restores the high affinity of the light-regulated sodium channel for cGMP, allowing the cell to adapt to background light (7).
The initial events in mollusks and arthropods are probably similar to those of vertebrates. However, whereas light activation of vertebrate photoreceptors leads to activation of cGMP-phosphodiesterase and generation of a hyperpolarizing response, activation of photoreceptors of invertebrates like Drosophila leads to stimulation of phospholipase C and generation of a depolarizing response (8). Cyclic GMP has also been implicated in modulating behavior in insects (9).
Cyclic GMP is also a second messenger in regulation mediated by natriuretic peptide hormones, but it is probably in the recently discovered nitric oxide (NO) regulation cascade that cGMP has the most unexpected role. Nitric oxide and atrial natriuretic peptide hormones play key roles in a number of neuronal functions, including learning, memory, and in blood circulation. Most experiments suggest that they exert converging actions by elevating of intracellular cGMP levels by activating soluble and membrane-bound guanylate cyclases. Cyclic GMP is the starting point for multiple signal transduction cascades, which are now beginning to be unraveled (10). In more than a quarter of a century since the discoveries of atrial granules and volume receptors in the heart atria, the search for natriuretic hormones has led to the isolation and identification of many atrial natriuretic factors (ANF) [(11), as a specific example see (12)]. In the heart, for example, ANF peptides are synthesized and stored in the Golgi apparatus of cardiac myocytes and are released in response to atrial wall stretch following acute plasma volume expansion and increased central blood volume. The mechanisms of the renal action of these potent natriuretic hormones are not yet completely unraveled. The renal hemodynamic, tubular, and adrenal, and systemic vascular effects are related to enhanced cGMP synthesis in specific medium-sized arteria, in the glomeruli and specific tubular segments, and in adrenal tissue. Specific ANF-binding sites have been detected in these target organs. A primary action of elevated cGMP levels is stimulating cGMP-dependent protein kinase) PKG), the major intracellular receptor protein for cGMP, which phosphorylates substrate proteins to trigger a regulation cascade (10).
Cyclic GMP-dependent protein kinases (PKG) also mediate some of the neuronal effects of cGMP (13), but unfortunately few PKG substrates are known in the brain. In striatonigral nerve terminals, for example, NO mediates phosphorylation of the protein phosphatase regulator, dopamine- and cyclic AMP-regulated phosphoprotein by PKG. PKG substrates are critically placed in the protein phosphorylation network and regulate protein phosphatases, intracellular calcium levels, and the function of many ion channels and neurotransmitter receptors. Nitric oxide is a signaling molecule in the nervous system of both mammals and insects. In contrast to classical transmitters, NO permeates membranes and acts on neighboring targets normally limited by diffusion barriers. This diffuse signaling is evolutionarily highly conserved. The NO forming enzyme, NO synthase, is present mostly in the nervous system, especially the brain. A soluble form of guanylate cyclase is the major target of NO action. Usually there is cellular separation of the release site and target site of NO, although exceptions to this rule exist.
As with cAMP, cGMP is important for memory in insects. In the honeybee, for example, the NO/cGMP system in the antennal lobes is implicated in the processing of adaptive mechanisms during chemosensory processing, and experimental data support a specific role of the NO system in memory formation (14).
Signal transduction in gastric and intestinal smooth muscle is mediated by receptors coupled via distinct G-proteins to various effector enzymes. Calcium is implicated in signal transduction in different ways according to the cell type (e.g., circular and longitudinal muscle cells). The initial steps involve Ca2+/calmodulin-dependent activation of myosin light-chain kinase and the interaction of actin and myosin. Relaxation is mediated by cAMP-and/or cGMP-dependent protein kinases. A specific cascade involves G-protein-dependent stimulation of Ca2+ influx, leading to Ca2+/calmodulin-dependent activation of a constitutive NO synthase in muscle cells that activates soluble guanylyl cyclase. The resulting activation of protein kinase A and PKG is jointly responsible for muscle relaxation (15).
Therefore, cyclic GMP is a secondary messenger that acts on targets that are sometimes similar to those of cAMP but proceed through a completely different cascade, in which the diffusible NO (and sometimes carbon monoxide) play a major role.
References
1. N. B. Bhatnagar, R. Bhatnagar, and T. A. Venkitasubramanian (1984) Biochem. Biophys. Res. Commun., 121, 634–640.
2. L. Stryer (1986) Ann. Rev. Neurosci. 9, 87–119.
3. T. Misaka, Y. Kusakabe, Y. Emori, T. Gonoi, S. Arai, and K. Abe (1997) J. Biol. Chem. 272, 22623-22629.
4. T. Nakamura and G. H. Gold (1987) Nature 325, 442–444.
5. J. T. Finn, M. E. Grunwald, and K. W. Yau (1996) Ann. Rev. Physiol. 58, 395–426.
6. M. Chabre, J. Bigay, F. Bruckert, F. Bornancin, P. Deterre, C. Pfister, T. Vuong, and D. Baylor (1988) Cold Spring Harbor Symp. Quant. Biol. 53 (Pt 1), 313–324.
7. D. Baylor (1996) Proc. Natl. Acad. Sci. USA 93, 560–565.
8. C. Zuker (1996) Proc. Natl. Acad. Sci. USA 93, 571–576.
9. K. Osborne, A. Robichon, E. Burgess, S. Butland, R. Shaw, A. Coulthard, H. Pereira, G. RJ, and M. Sokolowski (1997) Science 277, 834–836.
10. S. M. Lohmann, A. B. Vaandrager, A. Smolenski, U. Walter, and H. R. DeJonge (1997) Trends Biochem. Sci., 22, 307–312.
11. H. J. Kramer and B. Lichardus (1986) Klinische Wochenschrift 64, 719–731.
12. L. R. Forte, X. Fan, and F. K. Hamra (1996) Am. J. Kidney Dis. 28, 296–304.
13. X. Wang and P. J. Robinson (1997) J. Neurochem. 68, 443–456.
14. U. Muller (1997) Prog. Neurobiol. 51, 363–381.
15. G. Makhlouf and K. Murthy (1997) Cell Signal 9, 269–276.
الاكثر قراءة في مواضيع عامة في الاحياء الجزيئي
اخر الاخبار
اخبار العتبة العباسية المقدسة
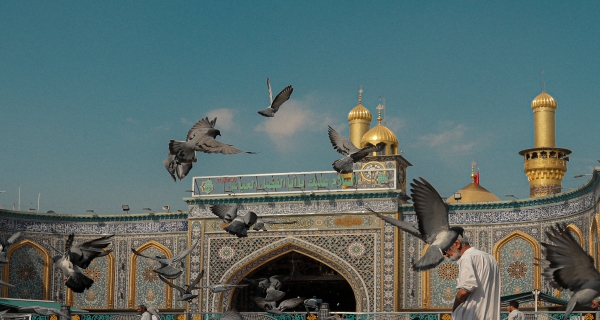
الآخبار الصحية
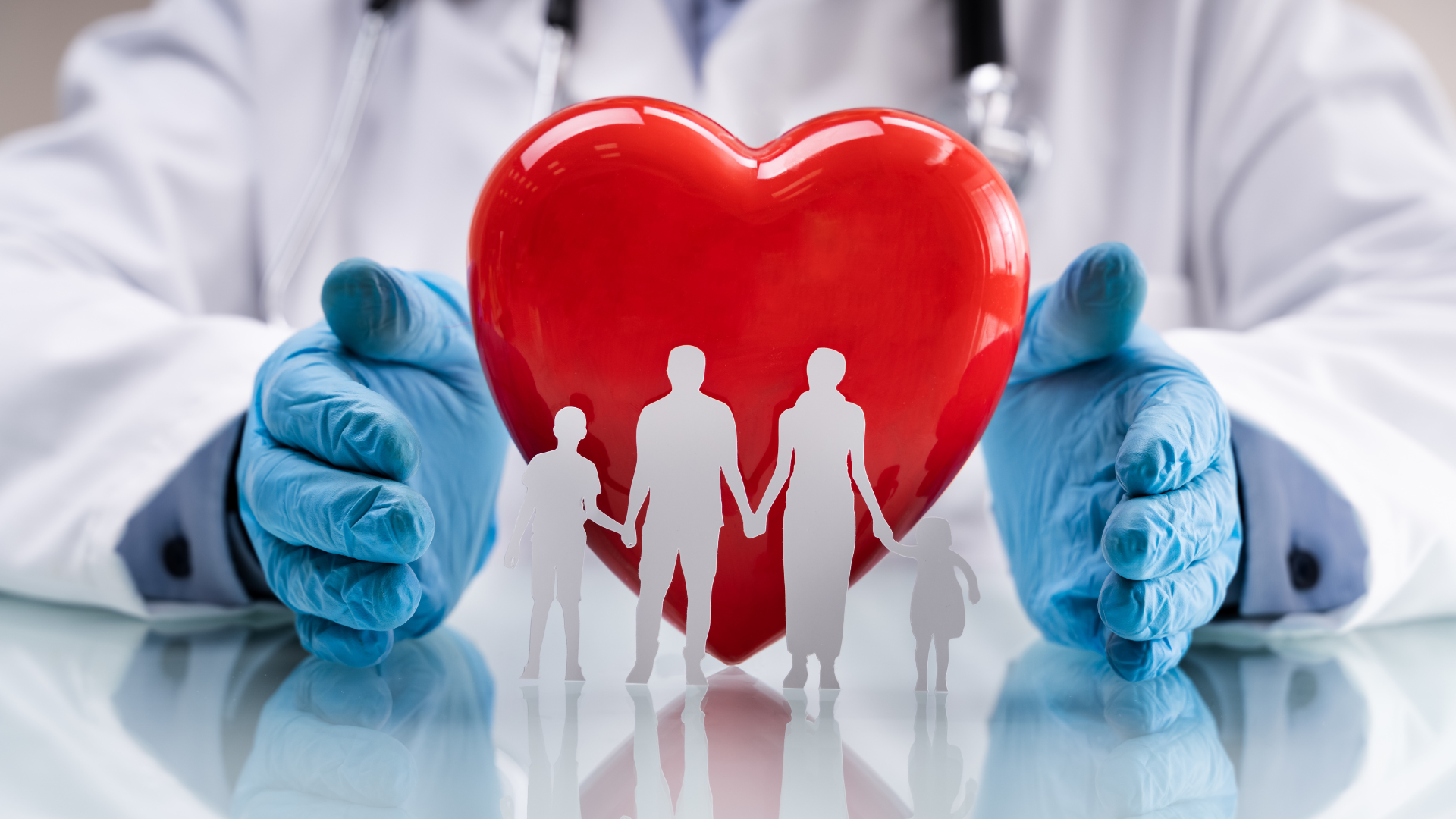