تاريخ الفيزياء
علماء الفيزياء
الفيزياء الكلاسيكية
الميكانيك
الديناميكا الحرارية
الكهربائية والمغناطيسية
الكهربائية
المغناطيسية
الكهرومغناطيسية
علم البصريات
تاريخ علم البصريات
الضوء
مواضيع عامة في علم البصريات
الصوت
الفيزياء الحديثة
النظرية النسبية
النظرية النسبية الخاصة
النظرية النسبية العامة
مواضيع عامة في النظرية النسبية
ميكانيكا الكم
الفيزياء الذرية
الفيزياء الجزيئية
الفيزياء النووية
مواضيع عامة في الفيزياء النووية
النشاط الاشعاعي
فيزياء الحالة الصلبة
الموصلات
أشباه الموصلات
العوازل
مواضيع عامة في الفيزياء الصلبة
فيزياء الجوامد
الليزر
أنواع الليزر
بعض تطبيقات الليزر
مواضيع عامة في الليزر
علم الفلك
تاريخ وعلماء علم الفلك
الثقوب السوداء
المجموعة الشمسية
الشمس
كوكب عطارد
كوكب الزهرة
كوكب الأرض
كوكب المريخ
كوكب المشتري
كوكب زحل
كوكب أورانوس
كوكب نبتون
كوكب بلوتو
القمر
كواكب ومواضيع اخرى
مواضيع عامة في علم الفلك
النجوم
البلازما
الألكترونيات
خواص المادة
الطاقة البديلة
الطاقة الشمسية
مواضيع عامة في الطاقة البديلة
المد والجزر
فيزياء الجسيمات
الفيزياء والعلوم الأخرى
الفيزياء الكيميائية
الفيزياء الرياضية
الفيزياء الحيوية
الفيزياء العامة
مواضيع عامة في الفيزياء
تجارب فيزيائية
مصطلحات وتعاريف فيزيائية
وحدات القياس الفيزيائية
طرائف الفيزياء
مواضيع اخرى
Nuclei and particles
المؤلف:
Richard Feynman, Robert Leighton and Matthew Sands
المصدر:
The Feynman Lectures on Physics
الجزء والصفحة:
Volume I, Chapter 2
2024-01-23
2035
What are the nuclei made of, and how are they held together? It is found that the nuclei are held together by enormous forces. When these are released, the energy released is tremendous compared with chemical energy, in the same ratio as the atomic bomb explosion is to a TNT explosion, because, of course, the atomic bomb has to do with changes inside the nucleus, while the explosion of TNT has to do with the changes of the electrons on the outside of the atoms. The question is, what are the forces which hold the protons and neutrons together in the nucleus? Just as the electrical interaction can be connected to a particle, a photon, Yukawa suggested that the forces between neutrons and protons also have a field of some kind, and that when this field jiggles it behaves like a particle. Thus, there could be some other particles in the world besides protons and neutrons, and he was able to deduce the properties of these particles from the already known characteristics of nuclear forces. For example, he predicted they should have a mass of two or three hundred times that of an electron; and behold, in cosmic rays there was discovered a particle of the right mass! But it later turned out to be the wrong particle. It was called a μ-meson, or muon.
However, a little while later, in 1947 or 1948, another particle was found, the π-meson, or pion, which satisfied Yukawa’s criterion. Besides the proton and the neutron, then, in order to get nuclear forces, we must add the pion. Now, you say, “Oh great! with this theory we make quantum nucleodynamics using the pions just like Yukawa wanted to do, and see if it works, and everything will be explained.” Bad luck. It turns out that the calculations that are involved in this theory are so difficult that no one has ever been able to figure out what the consequences of the theory are, or to check it against experiment, and this has been going on now for almost twenty years!
So, we are stuck with a theory, and we do not know whether it is right or wrong, but we do know that it is a little wrong, or at least incomplete. While we have been dawdling around theoretically, trying to calculate the consequences of this theory, the experimentalists have been discovering some things. For example, they had already discovered this muon, and we do not yet know where it fits. Also, in cosmic rays, a large number of other “extra” particles were found. It turns out that today we have approximately thirty particles, and it is very difficult to understand the relationships of all these particles, and what nature wants them for, or what the connections are from one to another. We do not today understand these various particles as different aspects of the same thing, and the fact that we have so many unconnected particles is a representation of the fact that we have so much unconnected information without a good theory. After the great successes of quantum electrodynamics, there is a certain amount of knowledge of nuclear physics which is rough knowledge, sort of half experience and half theory, assuming a type of force between protons and neutrons and seeing what will happen, but not really understanding where the force comes from. Aside from that, we have made very little progress. We have collected an enormous number of chemical elements. In the chemical case, there suddenly appeared a relationship among these elements which was unexpected, and which is embodied in the periodic table of Mendeleev. For example, sodium and potassium are about the same in their chemical properties and are found in the same column in the Mendeleev chart. We have been seeking a Mendeleev-type chart for the new particles. One such chart of the new particles was made independently by Gell-Mann in the U.S.A. and Nishijima in Japan. The basis of their classification is a new number, like the electric charge, which can be assigned to each particle, called its “strangeness,” S. This number is conserved, like the electric charge, in reactions which take place by nuclear forces.
Table 2–2
Elementary Particles
In Table 2–2 is listed all the particles. We cannot discuss them much at this stage, but the table will at least show you how much we do not know. Underneath each particle its mass is given in a certain unit, called the MeV. One MeV is equal to 1.783×10−27 gram. The reason this unit was chosen is historical, and we shall not go into it now. More massive particles are put higher up on the chart; we see that a neutron and a proton have almost the same mass. In vertical columns we have put the particles with the same electrical charge, all neutral objects in one column, all positively charged ones to the right of this one, and all negatively charged objects to the left.
Particles are shown with a solid line and “resonances” with a dashed one. Several particles have been omitted from the table. These include the important zero-mass, zero-charge particles, the photon and the graviton, which do not fall into the baryon-meson-lepton classification scheme, and also some of the newer resonances (K∗, ϕ, η). The antiparticles of the mesons are listed in the table, but the antiparticles of the leptons and baryons would have to be listed in another table which would look exactly like this one reflected on the zero-charge column. Although all of the particles except the electron, neutrino, photon, graviton, and proton are unstable, decay products have been shown only for the resonances. Strangeness assignments are not applicable for leptons, since they do not interact strongly with nuclei.
All particles which are together with the neutrons and protons are called baryons, and the following ones exist: There is a “lambda,” with a mass of 1115 MeV, and three others, called sigmas, minus, neutral, and plus, with several masses almost the same. There are groups or multiplets with almost the same mass, within one or two percent. Each particle in a multiplet has the same strangeness. The first multiplet is the proton-neutron doublet, and then there is a singlet (the lambda) then the sigma triplet, and finally the xi doublet. Very recently, in 1961, even a few more particles were found. Or are they particles? They live so short a time, they disintegrate almost instantaneously, as soon as they are formed, that we do not know whether they should be considered as new particles, or some kind of “resonance” interaction of a certain definite energy between the Λ and π products into which they disintegrate.
In addition to the baryons the other particles which are involved in the nuclear interaction are called mesons. There are first the pions, which come in three varieties, positive, negative, and neutral; they form another multiplet. We have also found some new things called K-mesons, and they occur as a doublet, K+ and K0. Also, every particle has its antiparticle, unless a particle is its own antiparticle. For example, the π− and the π+ are antiparticles, but the π0 is its own antiparticle. The K− and K+ are antiparticles, and the K0 and K̅0. In addition, in 1961 we also found some more mesons or maybe mesons which disintegrate almost immediately. A thing called ω which goes into three pions has a mass 780 on this scale, and somewhat less certain is an object which disintegrates into two pions. These particles, called mesons and baryons, and the antiparticles of the mesons are on the same chart, but the antiparticles of the baryons must be put on another chart, “reflected” through the charge-zero column.
Just as Mendeleev’s chart was very good, except for the fact that there were a number of rare earth elements which were hanging out loose from it, so we have a number of things hanging out loose from this chart—particles which do not interact strongly in nuclei, have nothing to do with a nuclear interaction, and do not have a strong interaction (I mean the powerful kind of interaction of nuclear energy). These are called leptons, and they are the following: there is the electron, which has a very small mass on this scale, only 0.510 MeV. Then there is that other, the muon, which has a mass much higher, 206 times as heavy as an electron. So far as we can tell, by all experiments so far, the difference between the electron and the muon is nothing but the mass. Everything works exactly the same for the muon as for the electron, except that one is heavier than the other. Why is there another one heavier; what is the use for it? We do not know. In addition, there is a lepton which is neutral, called a neutrino, and this particle has zero mass. In fact, it is now known that there are two different kinds of neutrinos, one related to electrons and the other related to muons.
Finally, we have two other particles which do not interact strongly with the nuclear ones: one is a photon, and perhaps, if the field of gravity also has a quantum-mechanical analog (a quantum theory of gravitation has not yet been worked out), then there will be a particle, a graviton, which will have zero mass.
What is this “zero mass”? The masses given here are the masses of the particles at rest. The fact that a particle has zero mass means, in a way, that it cannot be at rest. A photon is never at rest, it is always moving at 186,000 miles a second. We will understand more what mass means when we understand the theory of relativity, which will come in due time.
Thus, we are confronted with a large number of particles, which together seem to be the fundamental constituents of matter. Fortunately, these particles are not all different in their interactions with one another. In fact, there seem to be just four kinds of interaction between particles which, in the order of decreasing strength, are the nuclear force, electrical interactions, the beta-decay interaction, and gravity. The photon is coupled to all charged particles and the strength of the interaction is measured by some number, which is 1/137. The detailed law of this coupling is known, that is quantum electrodynamics. Gravity is coupled to all energy, but its coupling is extremely weak, much weaker than that of electricity. This law is also known. Then there are the so-called weak decays—beta decay, which causes the neutron to disintegrate into proton, electron, and neutrino, relatively slowly. This law is only partly known. The so-called strong interaction, the meson-baryon interaction, has a strength of 1 in this scale, and the law is completely unknown, although there are a number of known rules, such as that the number of baryons does not change in any reaction.
Table 2–3
Elementary Interactions
* The “strength” is a dimensionless measure of the coupling constant involved in each interaction (∼ means “of the order”).
This then, is the horrible condition of our physics today. To summarize it, I would say this: outside the nucleus, we seem to know all; inside it, quantum mechanics is valid—the principles of quantum mechanics have not been found to fail. The stage on which we put all of our knowledge, we would say, is relativistic space-time; perhaps gravity is involved in space-time. We do not know how the universe got started, and we have never made experiments which check our ideas of space and time accurately, below some tiny distance, so we only know that our ideas work above that distance. We should also add that the rules of the game are the quantum-mechanical principles, and those principles apply, so far as we can tell, to the new particles as well as to the old. The origin of the forces in nuclei leads us to new particles, but unfortunately, they appear in great profusion and we lack a complete understanding of their interrelationship, although we already know that there are some very surprising relationships among them. We seem gradually to be groping toward an understanding of the world of subatomic particles, but we really do not know how far we have yet to go in this task.
الاكثر قراءة في فيزياء الجسيمات
اخر الاخبار
اخبار العتبة العباسية المقدسة
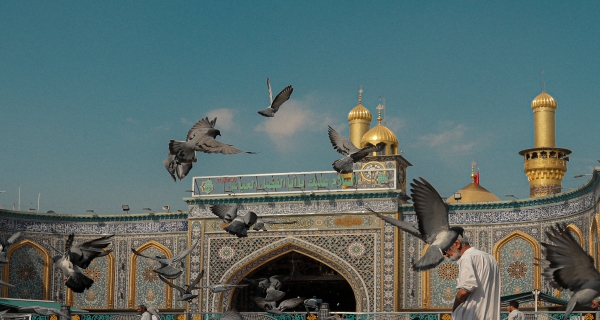
الآخبار الصحية
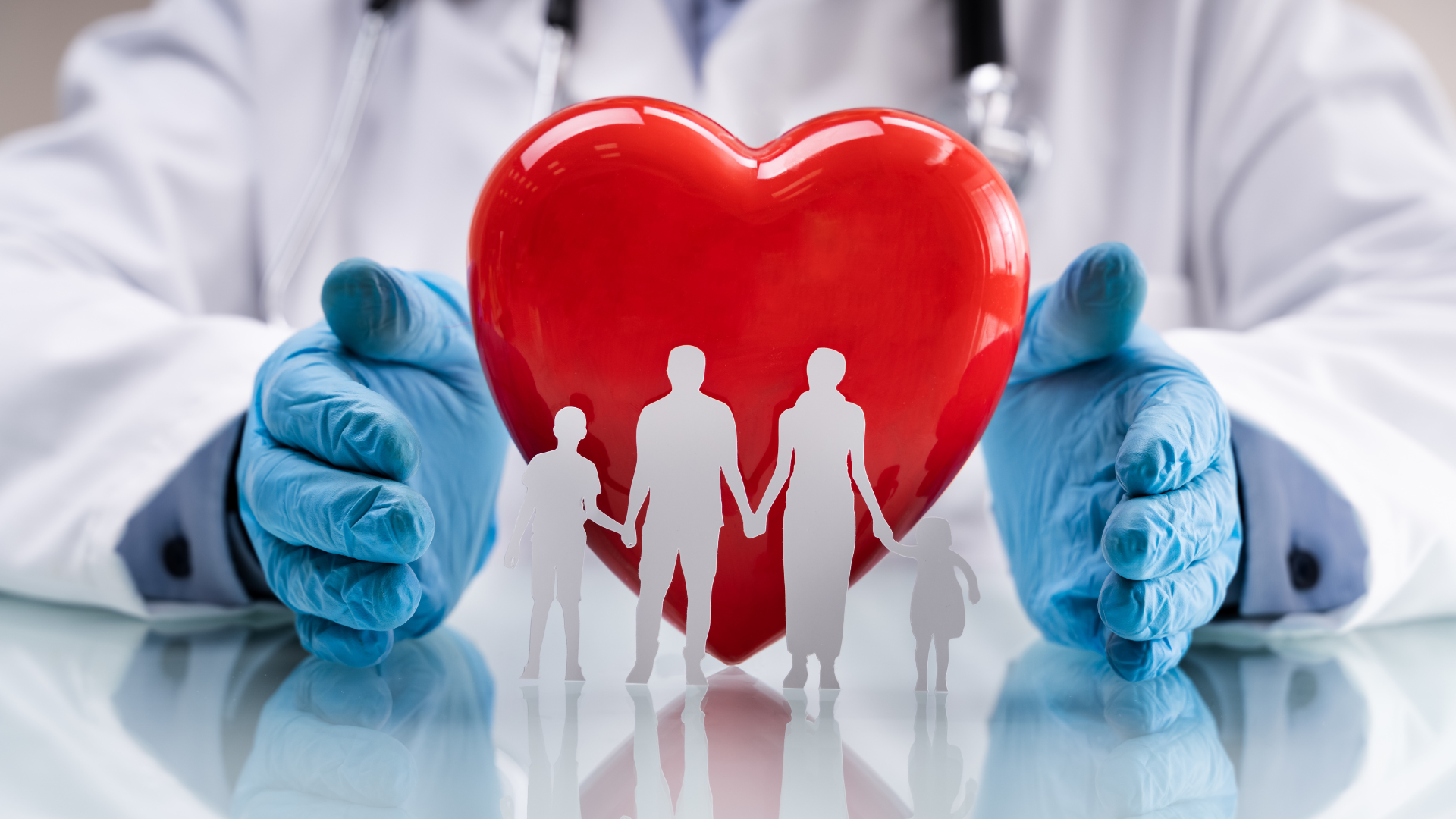